Background
Gastrointestinal hormones (GIH) have putative roles on physiological and biological reactions, companion enzyme activity, nutrient digestion, and meat quality [1,2], resulting in a higher level of humans’ health as consumers of meat. Cholecystokinin (CCK), secretin, and ghrelin are well-known GIH involved in several enzymes interrelation activities [3], many digestive processes [4], and in mechanisms which lead to higher meat quality. However, an understanding of the roles of the aforementioned hormones without considering the other factors that affect beef quality, amino acids (AA) [1], and their effects on GIH changes is imperfect, and needs further clarification.
L-tryptophan (L-T) as an essential component of protein synthesis is involved in the formation of serotonin and melatonin (MEL) [2,5,6], which has been reported to modulate the structural and functional composition of the intestinal microbiome in broiler chicks [7] and weaned piglets [4,8]. It has also been demonstrated that administration of L-T stimulates an increase of MEL synthesis in mice [9]. Both L-T and MEL were closely related to CCK, an endocrine hormone that can eventually improve feed digestion [1,8]. L-T deficiency may have a negative impact on food consumption and growth in monogastric animals [1,10,11]. In humans, it has been reported that intravenous administration of L-T increases prolactin and growth hormone in healthy subjects [12]. Additionally, an increase of MEL and ghrelin by L-T can stimulate the secretion of CCK [9,13,14]. Despite these important effects on GIH, the impact of L-T, which nutrients regulate GIH and MEL secretions, are largely unknown. Moreover, original research has been using laboratory animals such as mice to expand the knowledge in nutritional and hormonal aspects for the ease of experiment, lower costs, and higher growth and reproduction rates, etc. However, in the case of GIH research, extending the results obtained using laboratory animals to poly-gastric animals has been restricted due to natural differences and various reactions of AA supplementation by GIH tracts and stimulators in different species. A paucity of knowledge exists regarding the effects of intravenous administration of L-T in poly-gastric animals particularly beef cattle. An investigation of this phenomenon has potential to provide insights into the hormonal consequences of L-T administration with various dosages. Therefore, in order to find out the effective dosage of L-T administration into the blood, this study was undertaken to determine if similar neuroendocrine effects to those elicited could be obtained by an intravenous administration of L-T in beef cattle. The results of this work have potential to be used widely by the animal scientist community and particularly in ruminant studies.
Materials and Methods
The experimental procedure and methods were approved by the Animal Welfare and Ethics Authority of Pusan National University, Myrang, Korea. Three Korean native steers [ave. body weight (BW) = 362 ± 23 kg], with catheters in their jugular veins, were used. Animal intravenous 14G catheters (large animal intravenous catheter, NIPRO, Osaka, Japan) were connected to three-way valves (3W-RC type, NIPRO, Osaka, Japan) and inserted into their jugular veins for the simultaneous administration of L-T and blood sampling. Animals were kept in individual cattle sheds with a fence of 4 × 5 m and rice hulls on a concrete floor. Water was provided ad libitum. Each route in the cattle shed was closed 1 day prior to the experiment to block out light, thus minimizing its effect on MEL secretion. The cattle were fed according to their daily nutrient requirements and daily gain based on the NIAS standard [15]. Basal feed included timothy hay and concentrated feed (Table 1) to meet the daily energy consumption requirements for Korean native steers [15] and was provided twice daily at 800 and 1,700 h. Proximal analyses were performed based on AOAC [16]. Tryptophan in the feed and its requirement is not given due to the limitations in assay procedure as the similar limitation analysis has been stated by the Nutrient Requirements of Beef Cattle [17].
The powder form of 100% L-T (Sigma Co, St. Louis, MO, USA; Assay Spec. ≥ 98% by TLC) was used. The powder was dissolved in sterile saline and injected into jugular vein according to the method described by Kasuya et al. [3]. The total amount of L-T administered was set as low L-T (i.e. 28.9 mg/kg BW) or high L-T (i.e. 57.8 mg/kg BW). Sterile saline (1 L) was used as a control. Completely dissolved solution was filtered to remove external pollutants during degradation. All equipment used to prepare L-T solution was sterilized before use.
Steers were freely assigned into a 3 × 3 Latin square design including the following treatments: i) control (basal diet + physiological saline); ii) low L-T (basal diet + 28.9 mg L-T/kg BW); iii) high L-T (basal diet + 57.8 mg L-T/kg BW). After an adaptation period (7 days), treatments were administered via the jugular vein using a peristaltic pump for a period of 2 h (1,200 to 1,400 h). The treatment dosage was determined based on individual body weight measured one day prior to the study.
Blood was obtained from the jugular vein at 60 min (1,100 h) before L-T administration, 0 min (1,200 h) before administration, and 30 min (1,230 h), 60 min (1,300 h), 90 min (1,330 h), 120 min (1,400 h), 150 min (1,430 h), 180 min (1,500 h), 240 min (1,600 h), and 300 min (1,700 h) after AA administration. Blood was moved to the serum tube (BD Vacutainer, BD, USA) for storage at 4°C immediately after sampling. Samples were centrifuged (2,000 × g, 15 min) to separate serum. The supernatant was added to a 1.5 ml tube and stored at −80°C before analysis.
Serum concentrations of ghrelin, secretin, and CCK-8 were measured by enzyme immunoassay using secondary antibodies for ghrelin (EK-031-30), secretin (EK-067-05), and CCK-8 (EK-069-04) (Phoenix Pharmaceuticals, USA) with a human melatonin ELISA Kit (RE54021) (IBL, Germany). Serum was prepared for analyses and to test the cross reactivity of standards in the kit using the method described by Lee et al. [2].
Results
In this study, intravenous administration of 28.9 mg/kg BW of L-T was increased (p < 0.05) MEL after 30 min compared to the control (Fig. 1). However, no significant difference (p > 0.05) was observed over the total period (average of total period) during the administration and up to 5 h after injection (Table 2). Intravenously administration of 57.8 mg/kg BW of L-T significantly increased (p < 0.05) MEL synthesis at 30 and 120 min post administration. MEL synthesis was also significantly increased (p < 0.05) both during and post L-T administration compared to that in the control group.
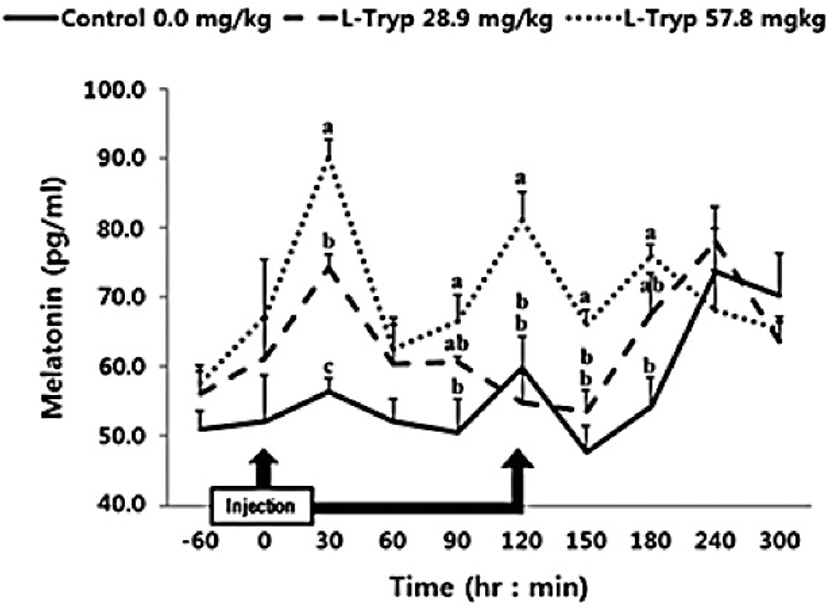
There was no increase (p > 0.05) in serum concentrations of CCK (Fig. 2) and secretin (Fig. 3) in the low L-T group. However, there was a significant increase (p < 0.05) in CCK and secretin in the high L-T group (Table 2).
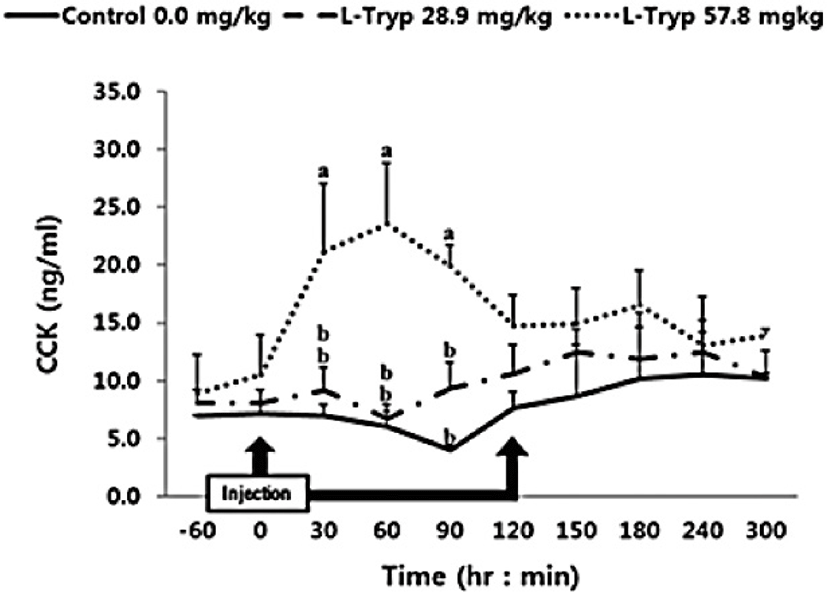
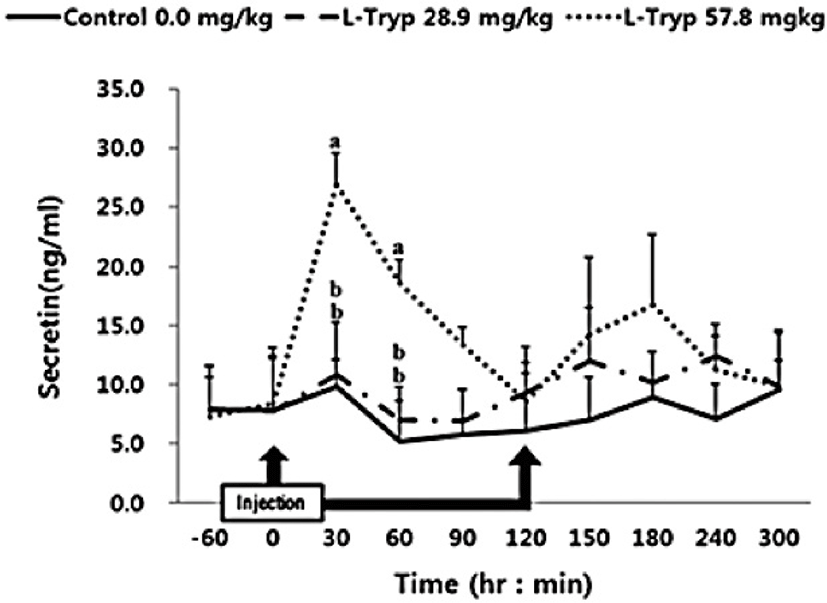
The concentration of serum ghrelin in the low and high L-T groups showed a downward compared to that of the control group (Fig. 4). A dose-dependent decrease in serum ghrelin was found following L-T administration, when samples were taken before and after administration. A significant decrease (p < 0.05) in serum ghrelin concentration was found in the high L-T group compared to the control group (Table 2).
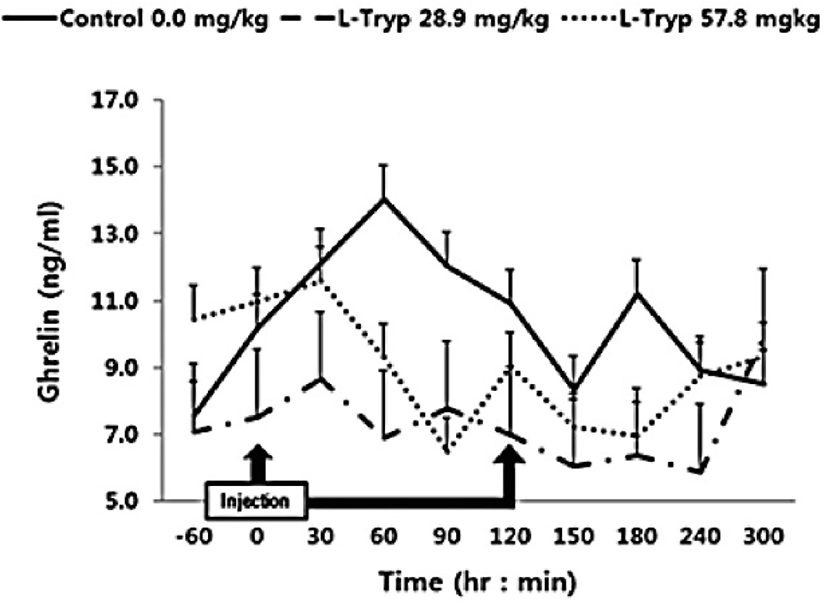
Discussion
Administration of L-T for both 28.9 and 57.8 mg/kg BW resulted in a dose-dependent increase in serum MEL concentration (Fig. 1 and Table 2). Jaworek et al. [16] reported that infusion of L-T (50 and 250 mg/kg BW) into the peritoneum or duodenum in mice increased the activity of plasma MEL. Administration of L-T for 28.9 mg/kg BW, caused an upward in serum concentration of GIHs including CCK and secretin compared to the control (Fig. 1 and Table 2). However, a dose-dependent increase in the treatment group was observed in response to the high L-T administration. Our finding complies with the findings by Leja-Szpak et al. [9] in mice, who reported an increase in serum MEL followed by administration of L-T. L-T and MEL have shown a positive relationship with CCK [4], which can ultimately increase the synthesis of pancreatic α-amylase to help digestion. Ghrelin, MEL, tryptophan, and other hormones can stimulate increasing CCK levels and the secretion of starch-degrading enzymes in monogastric [8,13]. The same phenomenon was approved in Hanwoo cattle in the present study. Therefore, increased MEL synthesis following L-T treatment might have increased the secretion of intestinal hormones including CCK.
Serum concentration of ghrelin was significantly decreased in the group administered with high L-T compared to the control in agreement with the study by Zhang et al. [17] in weaned pigs who reported higher plasma ghrelin in response to dietary supplementation of L-T [1]. Furthermore, L-T supplementation stimulated an increase in secretion of serotonin in the central nervous system [18–19]. Kasuya et al. [3] reported that L-T could stimulate 5-hydroxytryptamine (serotonin) secretion. However, treatment of the hypothalamic nervous structure of mice with ghrelin inhibited the secretion of 5-hydroxytryptamine [20]. To date, mechanisms behind modulation of blood ghrelin by L-T remained unclear. In general, the secretion of CCK is stimulated by the degradation of chain peptides and small molecules that enter the duodenum [21] and thus affect the meat production and quality. Secretion of CCK is related to the uptake of chained AA [22]. Furthermore, induction of endogenous MEL by L-T strongly stimulates the secretion of pancreatic proteins [9,16]. Leja-Szpak et al. [9] reported that administration of the L-T to mice can affect the synthesis of the pineal hormone MEL. Therefore, since both L-T and MEL are closely associated to the intestinal hormone CCK, the production of pancreatic α-amylase can be increased and thus be a theme of future in-vivo studies.
This study improved our understanding of how GIHs increase due to L-T administration as a source of AA. The results have a potential to be a theme of future ruminant studies aiming at maximizing of meat quality by dietary implication of L-T to safely passing from the rumen (encapsulating L-T). Collectively, this study endorsed the previous findings in laboratory and or monogastric animals with poly-gastric animals by which the effective dose of L-T found to be 57.8 mg L-T/kg BW, when administered intravenously. However, the oral rate of L-T, in practice, to achieve the same effective dose, need further research. Given the absorption rate of L-T via GIT (~72%), future studies can be designed to employ encapsulated L-T for oral use to investigate if similar results could be attained.