Background
Ruminants can digest high amounts of starch. However, since most feed contains over 60% starch, it is inevitable that some of the starch in feed will remain undigested [1]. In general, ruminants digest 78% to 90% of their starch intake [2]. On average, 35% to 60% of the starch entering the small intestine is decomposed, but 35% to 50% of the digestive food exiting the small intestine is digested in the large intestine [2]. However, the digestion rate of the large intestine is low, and the digested food is only related to survival of microbes in the large intestine, but is known to have a low relationship with the starch utilization of ruminants [1]. Thus, the digestion rate of the small intestine should be maximized to increase the utilization. In general, since the digestion process of ruminants is very complex, and many methods of increasing the production efficiency through starch are still available, we feel it necessary to accurately predict the digestion process of the small intestine and establish the most efficient method of starch digestion.
Taniguchi et al. [3] proposed that it is possible to improve the starch digestion in the small intestine of ruminants by means of protein. Richard et al. [4] reported that the starch digestion rate in the small intestine of steers together with four stomachs is increased by the injection of casein. This finding proves that in particular, the control by soluble proteins affects the secretion and activity of α-amylase in the small intestine, ultimately affecting the starch digestion.
Whey protein (WP) is a byproduct of the manufacturing process of cheese with a high content of soluble lactose (37% to 72%) [5,6]. WP can increase the entities of protozoa, which increases the utilization of carbohy-drates in ruminant feeds [7–9]. These protozoa indirectly improve the decomposition of significant amounts of fiber in the feed [10–12] and increases the rumen fermentation and the microbial synthesis of protein [13]. According to earlier study, WP increases the concentration of butyric acid and milk fat precursors which ferment carbohydrate in the rumen of milk cows [14]. The addition of WP to feed based on grain and grass [15] or low-quality hay [6] was reported to increase the weight and organic intake of beef calves. In the paper by Miron et al. [16], it was claimed that this is because the inflow of WP with ample lactose into the rumen increases the creation of bacteria and protozoa which use WP as an energy source, helping decompose the cell wall with a high ratio of structural carbohydrate. In addition, WP can change the kind, activity, or environment of microbes [6].
However, the previous research conducted on the utilization of WP has mostly focused on the characteristics of rumen fermentation. Thus far, there have been few papers related to the starch digestion in the small intestine after the rumen. Therefore, the objective of this research on Korean native steers is to investigate the effect of the oral administration of WP, a soluble protein, on the ruminal fermentation and in-blood secretion of gastrointestinal (GI) hormones, which are related to starch digestion in the small intestine, and the activity of pancreatic α-amylase in the small intestine, and then confirm the relationship of starch digestion in the small intestine.
Materials and Methods
This research used four Korean native steers (average posted weight 511 ± 17.2 kg) fitted with rumen and duodenal cannulas. Under general local anesthesia, these animals were surgically fitted with cannulas at the rumen (Bar Dia-mond™, Inc., Parma, Idaho, USA) and the duodenum (Simple T shaped type; Bar Diamond™, Inc., USA) so that the digestive food and the duodenal fluid could be collected from the rumen and the duodenum. The specimen animals were individually experimented on in the cattle shed with a fence of 3.0 × 3.2 m and a concrete floor where temperature and light are controlled (at 23°C, lights on for 16 h, lights out for 8 h), and water and a small amount of salt block were provided freely for the entire period. The weight of each animal was measured prior to the treatment period, and TMR (9 kg/d) was supplied as a basal feed twice a day at 9:00 AM and 5:30 PM. For the intake of metabolic energy, 1.2 times the maintenance requirement for a Korean native steer [17] was supplied, and as a marker for measurement of the content of digestive food, chromium oxide (Cr2O3) was added in the amount of 0.2% to the concentrated feed. The feed used in this research was WP (100% Any WP, optimum nutrition, USA) composed of 100% protein (protein: ≥ 85.7%; ash: ≤ 2.1%; moisture: ≤ 1.9%). The chemical compositions of WP and TMR used are given in Table 1. The study was authorized and undertaken in accordance with the ethics requirements of the Official Ethical Committee of Pusan National University (PNU 2015-0065).
1) Grobig DC provided following nutrients per kg of diet: 2,650,000 IU of vitamin A, 530,000 IU of vitamin D3, 1,050 IU of vitamin E, 10,000 mg of nicotinic acid, 13,200 mg of Fe, 4,400 mg of Mn, 4,400 mg of Zn, 2,200 mg of copper, 440 mg of iodine, 440 mg of cobalt. TMR, total mixed ration; DM, dry matter; CP, crude protein; EE, ether extract; CF, crude fiber; Ca, calcium; P, phosphorus.
The beef steers were arranged randomly as the treatment group under the 2 × 2 replicated crossover method. For 11 days prior to the treatment, only the basal feed was supplied, to provide a feed adaptation period. After the adaptation, the steers fed a basal feed (control; TMR 9 kg/d) or the basal feed with WP (400 g/d) during the experimental periods. The WP group was treated for 14 days per period for 2-period experimentation. All the amounts in corporation of WP to TMR were set by converting the individual weight measured 1 day prior to each experimental period.
Basal diet (500 g) was collected twice during each period, and then all the samples collected during the entire experimental period were mixed for application to the analysis. The samples were pulverized using a Wiley mill (Thomas Scientific Mode1 4, New Jersey, USA). Only the samples passed through the 1 mm net were used to analyze the moisture, crude protein, and ash using the general methods of AOAC [18], and starch was analyzed using the previous method [19].
For analysis of GI hormones and metabolites, blood samples were collected at 8:30 (−30), 10:30 (90), 13:30 (270), and 16:30 (450) on day 14, the last day of experimentation for each period. The 8:30 blood collection was conducted 30 mins prior to the morning feed supply. 20 mL of blood was collected from the jugular vein to pour 10 mL into each 10 mL serum tube (BD Vacutainer, BD, USA). In order to obtain serum at a refrigerated state of 4°C at the same time as the storage, centrifugal separation (1,500 × g, 15 min) was immediately performed. The upper layer liquid of serum was separated into 1.5 mL tubes for storage at −80°C prior to the analysis.
For analysis of the starch digestion rate of each GI tract, 250 mL of the contents which flow into the duodenum through duodenal cannula was collected at 8:30 (−30), 12:00 (180), 15:00 (360), at 18:00 (540) on day 12 during each period and 8:30 (−30), 10:30 (90), 13:30 (270), and at 16:30 (450) on day 13 during each period, was freeze-dried at −20°C (Bodiro Programmable Freeze dryer, Ilshin Lab. Co., Ltd., Gyeonggi-do, Korea), and then was pulverized to 1 mm using the disc mill (Model BM-D 100, Disc mill, McCoy Corp., San Marcos, TX). After the pulverization, all samples collected regardless of the collection time for each period were mixed for analysis of DM, CP, Ash, Starch, and Cr.
For analysis of α-amylase activity in the duodenal fluid, 50 mL of duodenal fluids was collected through the cannula installed at the duodenum at 8:30 (−30), 10:30 (90), 13:30 (270), and 16:30 (450) on day 14 of each period, and then frozen at −80°C prior to the analysis.
For analysis of the starch digestion rate for each GI tract, 300 mL of rumen fluids was sampled at 8:30 (−30), 10:00 (60), 12:00 (180), and 14:00 (300) on day 14 of each period. Immediately after the sample collection, the contents were filtered through 4-layer gauze to remove large particles from the sample, and then pH was measured using the pH meter (SevenEasy™ pH meters Mettler-Toledo AG 8603, Schwerzenbach, Switzerland). After pH measurement, they were pretreated with ammonia for analysis of volatile fatty acids (VFA), and then stored in frozen state (−70°C) in a deep freezer prior to the analysis.
Feces (300 g) was sampled at the same time as duodenal fluids using the retro-vaginal technique. All the sampled feces were dried at 70°C in the hot air circulation dryer (Vision scientific Co. Ltd., Daejeon-si, South Korea) for over 72 hr, and then the weight was measured. After the dried samples were pulverized to 1 mm using the disc mill (Model BM-D 100, Disc mill, McCoy Corp., San Marcos, TX), all samples collected regardless of the collection time of each period were mixed to take a sample for analysis of DM, CP, Ash, Starch, and Cr.
The volatile fatty acid in the rumen was analyzed using the method of Erwin et al. [20], as follows: As the pretreatment process for measurement of VFA, 1 mL of rumen fluids was sampled to put it into a 1.5 mL micro tube, and then 0.01 mL of HgCl2 was added to stop the activity of microbes. After putting 0.2 mL of H3PO4 into the mixed fluid in order to remove the microbial protein, 0.04 mL of pivalic acid, the internal standard for analysis of Gas chromatography (GC), was added. Then, the mixed fluid was stored in a frozen state (−70°C) in a deep freezer until the analysis. Next, the sample was melted at room temperature for the centrifugal separation at 5,300 × g for 5 min. The upper layer fluid was taken for analysis with GC (VARIAN model CP-3800, CA, USA). VFA standard solution was prepared as follows: After massing 100 mL of distilled water, 0.35 mL of acetate, 0.15 mL of propionate, 0.05 mL of isobutyrate, 0.1 mL of butyrate, 0.05 mL of isovalerate, 0.05 mL of valerate, and 5 mL of standard solution containing 0.2 mL of pivalic acid were added. 1 mL of the mixed solution was taken into a GC vial for use as VFA standard. The content of VFA in samples was measured in comparison with the calibration curve of VFA standard solution.
The serum concentration of ghrelin, secretin, and cholecystokinin (CCK)-8 was measured using such enzyme immunoassay methods as ghrelin (EK-031-30), secretin (EK-067-05), and CCK-8 (EK-069-04; Phoenix Pharmaceuticals Inc., USA) using a secondary antibody-coated plate. The immunoassay of ghrelin, secretin and CCK-8 in steer serum was performed in the same manner as in our previous research [21–23].
The α-amylase activity of the duodenal fluid secreted from the exocrine pancreas was measured by using En-zyChrom™ α-amylase Assay Kit (ECAM-100, BioAssay Systems, CA, USA).
The analysis of the nutrient digestion rate for each GI tract was performed using the anthrone method [24], which develops color with anthrone by using D-glucose as a standard reagent, and the analytical method of McCready et al. [19]. To describe it briefly, the GI fluid stored in a frozen state was melted at room temperature, and then pulverized below 0.5 mm under ice using a homogenizer. After the pulverization, the fluid was filtered using 2-layers of gauze (below-0.5 mm sieve), and 0.2 g of the sample was put into a 50-mL falcon tube (error allowance: 0.2 ± 0.001 g). To the sample of 0.2 g, 2 to 3 drops of 80% ethanol were added to wet the sample, and then 5 mL of distilled water was added. 25 mL of 80% ethanol stored at 75°C was added to the sample, followed by mixing. After it was left for 5 min, the sample was centrifuged at 980 × g for 10 min (1st centrifugal separation). After the centrifugal separation, the supernatant solution was discarded, and 30 mL of 80% ethanol at 75°C was added to the sample. After the well-mixed solution was left for 5 min, another centrifugal separation was conducted at 980 × g for 10 min (2nd centrifugal separation). 1 mL of the supernatant solution was taken to develop color in 5 mL of anthrone solution (solution prepared by dissolving 0.1 g of anthrone into 100 mL of the sulfuric acid solution, which is prepared by adding distilled water to 760 mL of sulfuric acid until the total solution reaches 1 L) and confirm whether or not a blue color is observed. At this time, if the blue color is developed, the centrifugal separation continues until the color disappears. After the centrifugal separation, the upper layer solution was discarded, and the residue was dried (40°C to 50°C, 60 min). The residue should be dried enough to remove the ethanol component completely. The temperature should not go over 60°C so as not to denature glucose and protein. After the drying, 5 mL of distilled water was added. To the mixture, 6.5 mL of 52% perchloric acid reagent was added every 5 min, for a total of 20 min. After the total mixture was mixed with 20 mL of distilled water, centrifugal separation was performed (980 × g, 10 min). After the centrifugal separation, 100 mL of the upper layer solution was filtered into a 100 mL volumetric flask. The processes after the drying were repeated once again. The residue of the leftover sample was filtered with distilled water to fill the flask exactly up to 100 mL (the last pretreatment process of sample). 1 mL of such standard solutions as 2.5 mL (25 μg/mL), 5 mL (50 μg/mL), 10 mL (100 μg/mL), 15 mL (150 μg/mL), and 20 mL (200 μg/mL) / 100 mL-distilled water and 1 mL of sample solution were put into a 20-mL tube, and then 5 mL of anthrone reagent was added. The process of color development was conducted at 100°C for 12 to 13 min. After the color development, the sample was quenched to room temperature, and the measurements were made with the spectrophotometer (630 nm). Prior to the measurement of all the samples, any one of them was diluted stepwise, and then compared with the standard solution to select the dilution ratio, which was then applied to all samples for analysis. The starch digestion rate was calculated by obtaining the D-glucose content in each digestive organ and then comparing it with Cr content. The D-glucose content of the feed was converted to the starch content.
A sample (2 g) was put into a porcelain crucible, 0.5 to 1 mL of No. 3 potassium phosphate solution (K3PO4), a chromium oxidant, was added to the sample depending on the state of the sample, and then the sample and the solution were mixed completely by stirring with a glass rod. The glass rod was washed with cotton wool, put into the porcelain crucible to pre-incinerate them together with the sample in the electric furnace (Muffle furnace, Model MF-64, HYSC, Seoul, South Korea), and then burned at 800°C for 50 min. After cooling to room temperature, the contents left in the crucible were eluted with distilled water into a 100-mL volumetric flask until the mixture reaches the mark line. Then, the mixture was left for 24 hr. Thereafter, the sample was filtered to measure chromium concentration with inductively coupled plasma.
The daily amount of the flowed nutrient (flow, g/d) for the daily amount of supplied chromium was obtained using the ratio of chromium to the amount of the nutrient in the contents of the samples collected from GI tract. The equation is as follows.
By substituting the amount of flow into the small intestine and amount of discharge into feces as obtained by the above equation into the equations below, the amount of nutrient digestion (Disappearance, g/d) and rate of nutrient digestion (Disappearance, %) for the rumen, lower digestion tubes, and each GI tract were obtained.
The analysis of metabolites in blood was performed using Toshiba Acute Biochemical Analyzer-TBA-40FR (Toshiba Medical Instruments, Tochigi-ken, Japan), a biochemical analyzer, to confirm in the serum if the biological metabolic index is changed in comparison with the control group.
Results
The rumen fermentation characteristics due to the addition of WP to the basal feed are given in Table 2. No significant difference between the groups in the pH of the rumen was observed (p > 0.05). In addition, there was no difference shown in total VFA, or in acetate, propionate, butyrate, and acetate/propionate ratio out of VFA (p > 0.05). However, the ratios of valerate and isovalerate out of VFA increased significantly in the WP group in comparison with the control group (p < 0.05).
The flow and digestion rate of crude protein for each area of the GI tract due to the addition of WP to the basal feed are given in Table 3. As shown in the Table, the crude protein intake of the WP group (1,304.1 g/d) was higher than that of the control group (973.1 g/d) (p < 0.05). However, the content of crude protein flowing into the small intestine showed only a difference of 94 g/d between the control group (485.1 g/d) and the WP group (579.1 g/d), which was insignificant (p > 0.05). The content of crude protein for discharge into feces also did not show a large difference between the control group (150.8 g/d) and the WP group (166.3 g/d). The amount of protein digestion in the rumen of the WP group (725.1 g/d) was higher than that of the control group (488.0 g/d) (p < 0.05). In the small intestine, however, the WP group (412.8 g/d) digested 78.6 g/d more than the control group (334.2 g/d), which is not significant (p > 0.05). Consequently, the crude protein digestion of the entire GI tract was higher to a statistically significant level in the rumen of WP group (p < 0.05).
The flow and digestion rate of starch for each area of the GI tract due to addition of WP to the basal feed are given in Table 4. The results showed no difference between the starch content for flow into the small intestine and the starch content in feces, and the digestion rate also showed no difference between the rumen and the duodenum (p > 0.05). However, the starch content flowing into the small intestine was 139.3 g/d more in the rumen of the WP group (704.4 g/d) than the control group (843.7 g/d). And amount of digestion (139.3 g/d) and the digestion rate (5.2%) also showed more increased values in the rumen of the WP group than the control group. However, the starch content in feces showed a difference of 47.2 g/d between the control group (152.8 g/d) and the WP group (105.6 g/d), which was smaller than that in the rumen, indicating that the starch utilization in the small intestine was significantly decreased by the addition of WP to basal feed in beef steers. This could be supported by the amounts of digestion in the small intestine of the control group (690.9 g/d) and the WP group (598.9 g/d). However, there was no significant difference (p > 0.05).
The relationship between GI hormone and α-amylase activity due to addition of WP to the basal feed are shown in Fig. 1 and Table 5. Overall, the addition of WP to the basal feed in this experiment showed no close relationship with ghrelin, CCK, and secretin, the GI hormones related to the secretion of enzymes, and the activity of α-amylase, a starch decomposition enzyme (p > 0.05). For GI hormones, the time change after the oral treatment also showed a similar trend to that of the control group, while for duodenal α-amylase activity, the WP group also did not show any significant difference from the control group (p > 0.05). In Table 5, which shows the average values of the entire experimental period, it can be seen that the WP group also did not show any effects on GI hormones and pancreatic external secretion.
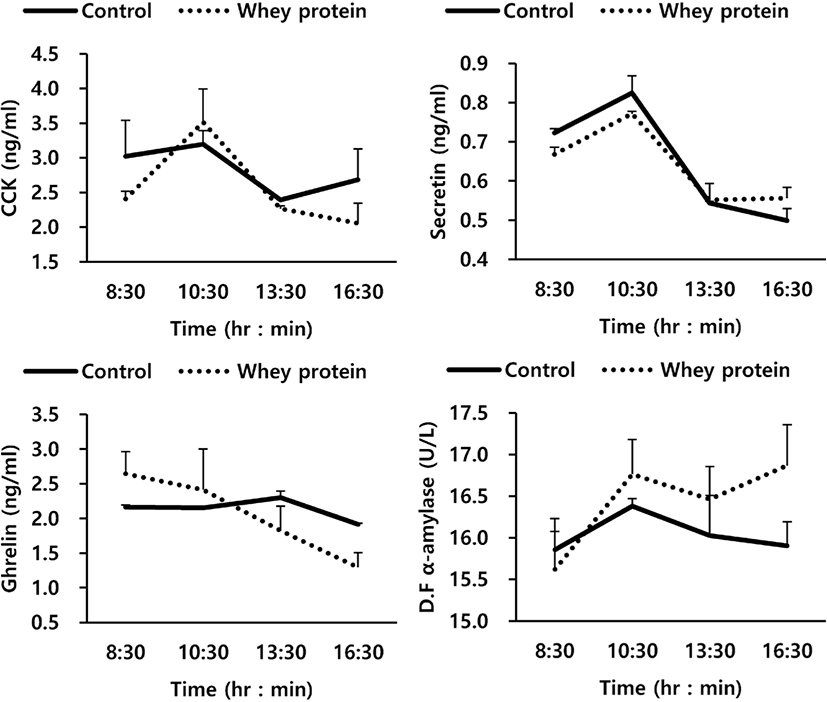
Meanwhile, according to the results for investigation of the change of metabolites in blood due to addition of WP (Fig. 2), BUN (Blood Urea Nitrogen) out of the protein metabolites increased more significantly in the WP group than in the control group (p < 0.01), but no difference was observed between the control group and the WP group for the other protein metabolites (total protein, albumin), lipid metabolites (triglyceride, total cholesterol), carbohydrate metabolites (glucose), and mineral metabolites (phosphorus, magnesium, calcium).
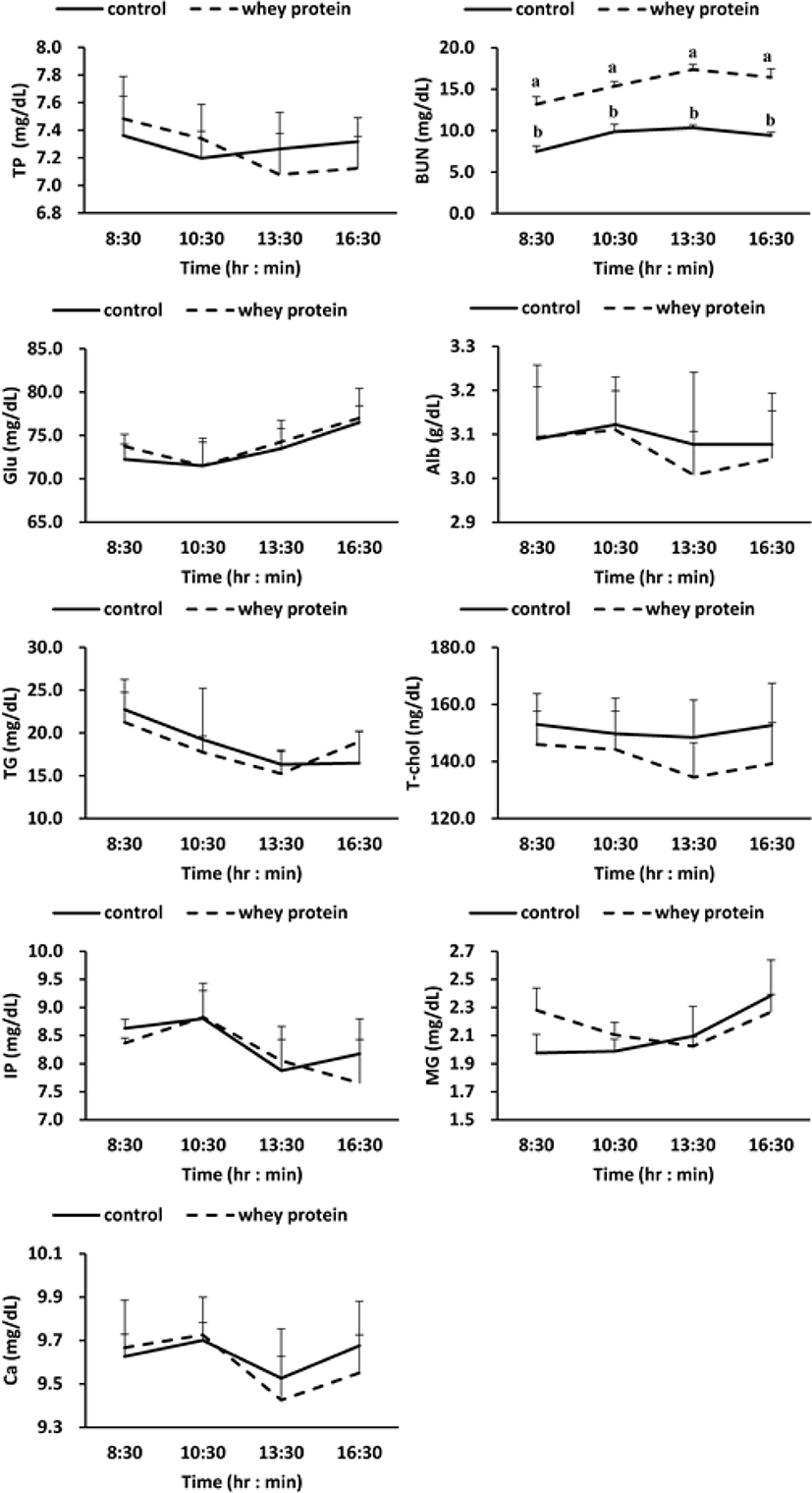
Discussion
The addition of WP to the animal feed did not affect the change of pH and total VFA, the rumen fermentation characteristics, but the concentration of valerate and isovalerate out of VFA concentrations increased significantly in the WP group (p < 0.05; Table 2). The increased supply of protein to ruminants was reported to increase branched-chain volatile fatty acids (BCVFA) [25,26]. According to the research findings of Ludden et al. [25] for different-level supply of CP content to sheep (0%, 13%, 15%, and 17%), as CP content increased, the ratios of valerate and isovalerate increased significantly (p < 0.05). The research of Ipharraguerre et al. [26] also reported that as the supply of CP to milk cows increased, the ratios of isovalerate and isobutyrate increased significantly (p < 0.05). In the rumen, BCVFA was reported to be created in the decomposition process of such branched-chain amino acids (BCAA) as valine, leucine, iso-leucine, and proline or used in re-synthesis of BCAA [27–29], and to increase the synthesis of rumen microbial protein and the digestion rate of structural carbohydrates and dried materials [30].
The results of the investigation of the flow and digestion rate of protein for each area of the GI tract due to the addition of WP to the basal feed (Table 3) show that while significantly more digestion was conducted in the rumen of the WP group than the control group (p < 0.05), no changes were observed in the small intestine (p > 0.05). Galloway et al. [6] proposed that WP could change the kind, activity, or environment of microbes. In ruminants, numerous studies have reported that WP promoted the creation of such microbes as bacteria and protozoa in the rumen [7–9], and increased the ruminal fermentation and the protein synthesis of microbes [13]. Since WP contains a lot of protein and lactose, the propagation of bacteria and protozoa, which use protein and lactose as an energy source, was reported to increase with the use of WP [16]. Eventually, the ruminal microbes propagated by the lactose contained in WP were confirmed to decrease the content of crude protein for flow into the small intestine by increasing the decomposition and utilization of WP crude protein.
The results of the investigation of the flow and digestion rate of starch for each area of GI tract due to the addition of WP to the basal feed (Table 4) also showed no difference in the starch content flowing into the small intestine, the starch content in feces, and the digestion rate between the rumen and the duodenum (p > 0.05), but showed that the use of starch in the entire GI tract tended to be increased by the addition of WP to the basal feed (p = 0.094). In addition, although there was no significant difference, the starch content flowing into the small intestine was used more in the rumen of the WP group (704.4 g/d) than the control group (843.7 g/d). Furthermore, the amount of digestion (139.3 g/d) and the digestion rate (5.2%) also showed more increased values in the rumen of the WP group than in the control group.
In general, the addition of WP to the basal feed of ruminants was reported to improve the decomposition of fiber in the rumen by increasing the population of ruminal protozoa [7–9], decreasing the amount of non-structural carbohydrates [6]. Additionally, this protozoa was reported to indirectly improve the decomposition of starch by assisting in decom-position of the cell wall of fiber with a high ratio of structural carbohydrates in the feed [10–12,16]. Thus, the addition of WP to the basal feed was confirmed to improve the starch decomposition in the rumen by assisting in the creation of ruminal microbes in the rumen, but had only a small influence on the starch digestion in the small intestine.
The results of the investigation of the relationship between GI hormones and α-amylase activity due to the oral treatment of WP (Fig. 1 and Table 5) show that the oral treatment of WP, overall, was not closely related to ghrelin, CCK, and secretin, the GI hormones related to the secretion of enzymes, or the activity of α-amylase, an enzyme for decomposition of starch (p < 0.05). α-Amylase is a starch decomposition enzyme secreted from the small intestine by stimulating the pancreatic cystocavitary cell with CCK and secretin, the GI hormones stimulated by amino acids / fatty acids flowed into the small intestine, and pH [31,32]. In particular, according to the findings of previous research using intact casein (IC), such GI hormones as CCK and secretin were confirmed to be increased significantly by peptide and soluble non-ammonia N (SNAN) out of the proteins flowed into the small intestine [21]. The reason for the difference between IC and WP in the secretion of internal-secretion hormones and pancreatic α-amylase may be due to a representative factor of the component difference between IC and WP. While IC is composed of α-casein 59% (α S1-, α S2-), κ-casein 15%, β-casein 38%, and γ-casein 3%, WP is composed of β-lactoglobulin 65%, α-lactalbumin 25%, bovine serum albumin 8%, and immunoglobulins 2%. In general, bacteria and protozoa which live in the rumen mentioned earlier use high amounts of the lactose of WP, which decreases the content of non-decomposed proteins (rumen undegradable protein, RUP) in the rumen. According to the paper by Bethard et al. [33], the supply of RUP to ruminants could improve the efficiency and growth of nitrogen utilization by increasing the content of amino acid and nitrogen (N) flowed into the small intestine. Such GI hormones as CCK and secretin were reported to be affected by amino acid and nitrogen flowed into the small intestine, particularly SNAN [4,34], but the addition of WP to the feed could not affect the crude protein flowed into the small intestine (Table 3). In addition, WP may be used as an energy source of ruminal microbes (protozoa, bacteria) to increase the population of ruminal microbes in the rumen. It is judged that while the ruminal microbes increased the utilization of protein in the rumen, they could not affect the stimulation of internal-secretion hormones for protein flowed into the small intestine.
In this research, the addition of WP to the basal feed increased the concentration of BUN out of the blood metabolites (Fig. 2). From this phenomenon, it is judged that the amino acid created by the decomposition of protein from the WP was deaminated by various enzymes into ammonia, which was then synthesized into urea in the liver through the urea cycle [35]. However, the addition of WP to the basal feed did not affect such other protein metabolites as total protein and albumin, mineral metabolites, lipid metabolites, and carbohydrate metabolites.
Thus, we considered that though WP-added supply may assist in ruminal fermentation due to the population increase of microbes in the rumen, it does not affect the secretion of hormones related to the starch digestion in the small intestine and the secretion of α-amylase in the pancreas, nor does it assist in starch digestion in the small intestine.