INTRODUCTION
Gut health plays a crucial role in determining the overall health and welfare of pigs and their production performance through nutrient utilization [1–5]. Therefore, maximizing intestinal function is fundamentally important for successful livestock farming. Gut health encompasses various physiological functions, including nutrient digestion and absorption, physical and chemical immune systems, and a balanced microbial ecosystem [5,6]. However, pigs may face gut-related issues due to their diet, management, and living environment, which are often exacerbated by modern pig production systems [7–9]. Therefore, there is a lot of interest and various approaches to manage and improve intestinal health problems, and feed additives using them are continuously provided [10,11].
A balanced microbiome is an essential component of a healthy gut [12]. Previous studies have shown that probiotics, which are live microorganisms that confer favorable health benefits to the host when administered in adequate amounts, are a category of feed additives that can be used to replenish the gut microbial population while restoring the host’s immune system, primarily to the microorganisms and to the host. We have ample evidence that it may help improve gut health due to its specific biochemical interactions with and systemic integration into the host biology [13–16].
The bacterial strains most often used as probiotics are Bacillus, Lactobacillus, Enterococcus, Streptococcus, Saccharomyces cerevisiae (S. cerevisiae), and Aspergillus spp. [17–19]. Unlike Lactobacillus and Enterococcus, S. cerevisiae is not a natural host of gut microorganisms in monogastric animals such as pigs. Thus, S. cerevisiae flows along the gastrointestinal tract (GIT) as alive and active without adhering to its walls [20]. Strains lacking the ability to adhere to the intestinal epithelium are effective as biological regulators, and their efficacy varies depending on their ability to form colonies through various mechanisms [21]. A synergistic effect can be obtained as complex probiotics by combining useful microorganisms and S. cerevisiae in the intestines (Table 1). It has been suggested that multiple strains of probiotics may be more effective than single strain probiotics due to their additive and synergistic effects [22,23], and many previous studies have demonstrated that dietary complex probiotic supplementation has growth-promoting effects in pigs [24,25].
Animal | Composition of strains | Effect of feeding | References |
---|---|---|---|
Weaned pigs |
B. subtilis
B. licheniformis S. cerevisiae |
Improved ADG Increased Lactobacilli |
[63] |
L. acidophilus
B. subtilis S. cerevisiae A. oryzae |
Improved growth performance (ADG and gain/feed) and nutrient digestibility (DM, energy) Increased Lactobacilli with decreased Clostridium spp. counts |
[60,61] | |
L. acidophilus
B. subtilis S. cerevisiae |
Improved ADG Increased Lactobacilli with decreased E. coli counts Increased villus height |
[62] | |
L. acidophilus
B. subtilis S. cerevisiae |
Better FCR with no differences in nutrient digestibility and fecal score Increased Lactobacilli |
[85] | |
E. faecium
B. subtilis S. cerevisiae |
Improved growth performance (ADG and gain/feed) and diarrhea score Increased production of VFAs (acetate and propionate) |
[59] | |
B. licheniformis
B. subtilis S. cerevisiae |
Improved IgG Increased Lactobacilli with decreased E. coli counts |
[86] | |
L. acidophilus
S. cerevisiae |
Improved growth performance (ADG and gain/feed) and diarrhea score (DM, energy) Increased Lactobacilli with decreased E. coli counts |
[92] | |
B. licheniformis
S. cerevisiae |
Improved villus height and villus height to crypt depth ratio in small intestine Enhanced intestinal barrier integrity |
[93] | |
Growing and finishing pigs |
L. acidophilus
B. subtilis S. cerevisiae |
Increased total anaerobic bacteria, Lactobacilli with decreased Clostridium spp. counts | [113] |
L. fermentum
S. cerevisiae B. subtilis |
Better FCR with no differences in nutrient digestibility | [85] | |
L. plantarum
B. subtilis S. cerevisiae |
No difference in growth performance and digestibility | [74] | |
S. cerevisiae
L. casei L. plantarum |
Increased drip and cooking loss Decreased juiciness and tenderness |
[110] | |
L. acidophilus
L. plantarum B. subtilis S. cerevisiae |
No difference in the growth performance | [67] |
B. subtilis, Bacillus subtilis; B. licheniformis, Bacillus licheniformis; S. cerevisiae, Saccharomyces cerevisiae; ADG, average daily gain; L. acidophilus, Lactobacillus acidophilus; A. oryzae, Aspergillus oryzae; DM, dry matter; E. coli, Escherichia coli; FCR, feed conversion ratio; E. faecium, Enterococcus faecium; VFA, volatile fatty acid; IgG, immunoglobulin G; L. fermentum, Limosilactobacillus fermentum; L. plantarum, Lactiplantibacillus plantarum; L. casei, Lacticaseibacillus casei.
A complex probiotic supplement contains various strains of beneficial microorganisms and is a health supplement. These strains have similar properties to the bacteria naturally occurring in the digestive system of humans or animals and can provide many health benefits. Wang et al. [26] reported that the administration of a complex probiotic supplement to nursing piglets improved their growth rate, enhanced nutrient digestibility, and had a positive effect on the microbial population of their feces as well as reducing odor emissions. Furthermore, Kang et al. [27] reported that the administration of probiotics not only improved nutrient digestibility leading to increased growth in pigs, but also positively modulated the microbial population within the intestinal tract. In practice, however, the effectiveness of complex probiotics is not always consistent, and the effectiveness of probiotics can be influenced by strain composition, dosage, feed formula, environment, sanitation, and age of the animal [28–30]. In this review, we will discuss the effect of a complex probiotic with S. cerevisiae, which is primarily used as a probiotic.
Yeasts are known to have soluble proteins, vitamin B complexes and minerals, unknown growth factors, and produce enzymes such as amylase, galactosidase and phytase [31,32]. Today, yeast is used in a variety of fermentation processes and plays a vital role in many industries, including food, beverage, pharmaceuticals, and commercial enzyme production [33]. Among them, it is attracting attention as probiotics for improving the intestinal environment, preventing and treating diarrhea, and its use is increasing in the livestock industry. A yeast additive commonly used in animal feed is S. cerevisiae, which is known to have positive effects on both ruminants and non-ruminants [30,32]. In ruminants, S. cerevisiae has been reported to increase feed efficiency and improve milk production and milk quality [34]. In non-ruminants, the addition of S. cerevisiae has been reported to improve piglet productivity [35–38] and increase resistance to enterotoxigenic Escherichia coli (ETEC) F4 or Salmonella infection [39,40]. These results suggest that S. cerevisiae improves fiber digestibility, produces antibacterial compounds, stimulates the immune system, inhibits the growth of pathogens, and improves intestinal morphology and structure in animals, ultimately contributing to improved productivity [21,33,41,42].
S. cerevisiae is effective as a biological regulator due to its strong acid resistance, excellent ability to reach the intestine, and ability to maintain activity without adhering to the intestinal wall [30]. In particular, S. cerevisiae has a proven immune modulatory ability [43–46]. Buts et al. [43] reported that including S. cerevisiae in pig feed has an impact on immune responses. Additionally, Qamar et al. [44] reported that S. cerevisiae in pig feed increases the activity of immunoglobulin M (IgM) and A (IgA) against pathogenic microorganisms, thereby enhancing mucosal immunity. It is understood that this reason is due to polysaccharides such as β-glucan and mannan-oligosaccharides in the cell wall of S. cerevisiae [47,48]. β-Glucan is believed to be associated with immune system stimulation and is thought to enhance immune responses [49–51]. Mannan in particular contributes to various biological functions by inhibiting pathogen attachment to the mucosal surface of the GIT, thereby aiding in infection prevention and maintaining intestinal health [50,52–55]. Additionally, the proteins present in the cell wall of S. cerevisiae interact with the pig’s intestinal environment, giving it properties that allow it to participate in cell signaling and immune system regulation in pigs [56,57].
These mechanisms collectively enhance gut development and function and regulate the balance of gut microbiota (Fig. 1). Additionally, organic acids such as lactic acid and acetic acid produced by the addition of yeast reduce intestinal pH, creating a more favorable environment for the innate gut microbiome and reducing the potential for pathogen colonization [58]. Therefore, the combination of intestinal beneficial bacteria and S. cerevisiae is expected to produce a synergistic effect as complex probiotics.
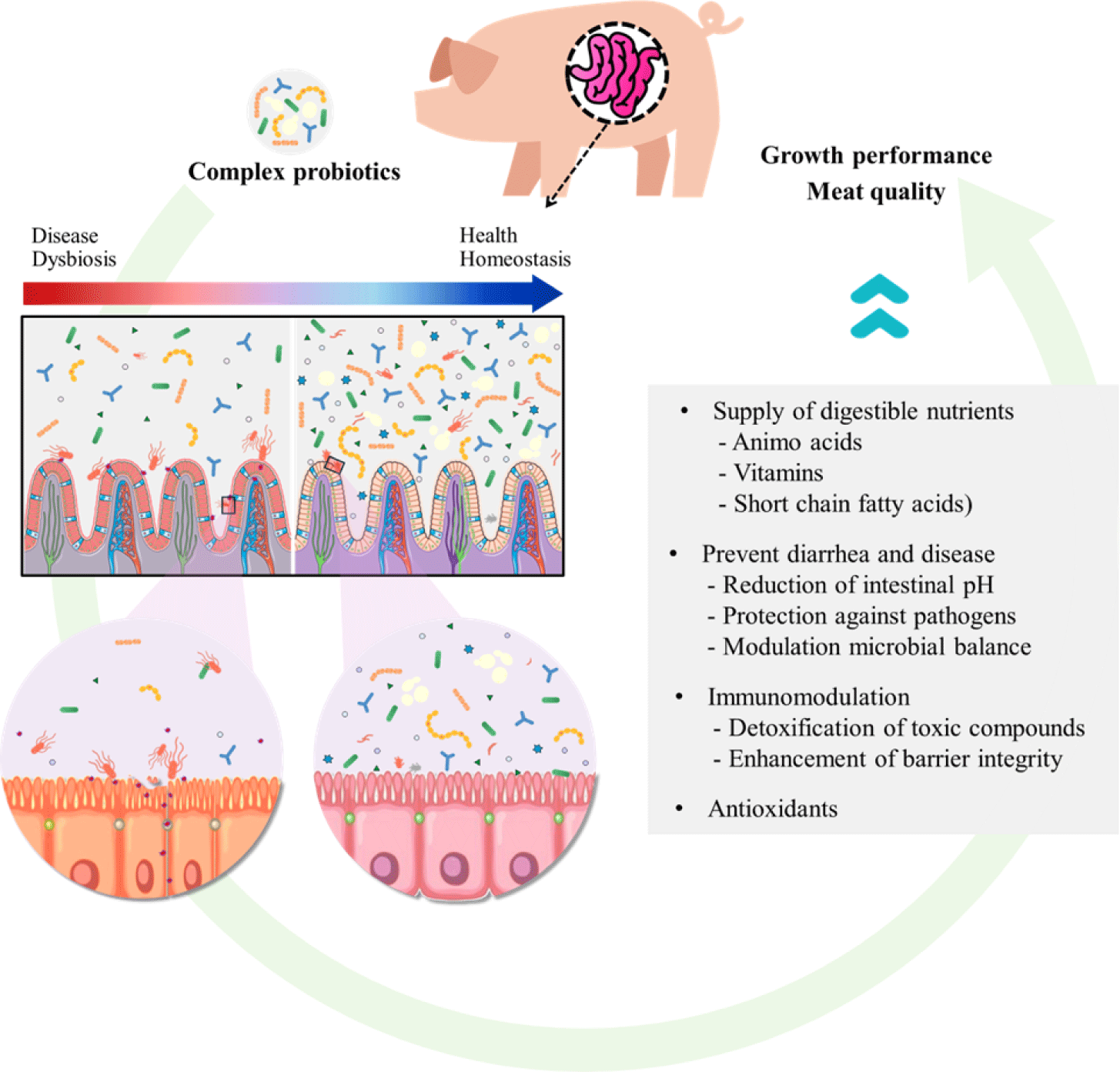
APPLICATIONS AND BIOLOGICAL FUNCTIONS OF MULTI-STRAIN PROBIOTICS CONTAINING SACCHAROMYCES CEREVISIAE
Previous studies have reported positive effects on growth when administering a complex probiotic containing S. cerevisiae to pigs (Table 1). Lu et al. [59] reported that feed efficiency and average daily gain (ADG) were significantly improved when pigs were fed complex probiotics for 3 weeks. Choi et al. [60–62] also found that the growth of piglets receiving the complex probiotic (L. acidophilus 4.0 × 108 CFU/g, B. subtilis 4.8 × 109 CFU/g and S. cerevisiae 1.0 × 107 CFU/g) improved to a similar level as the antibiotic-treated group. Sampath et al. [63] also reported improved growth in piglets fed a low-density diet when supplemented with the complex probiotic (1.5 × 109 CFU/g of Bacillus subtilis ms1, 1.5 × 109 CFU/g of B. licheniformis SF5-1 and 1.5 × 109 CFU/g of S. cerevisiae). Previous studies of growing and finishing pigs also reported that supplementing pigs with a complex probiotic improved ADG and feed efficiency [18,64,65]. Wang et al. [66] reported that supplementing growing pigs fed a low-density diet with a complex probiotic (1.5 × 109 CFU/g of B. subtilis ms1, 1.5 × 109 CFU/g of B. licheniformis SF5-1 and 1.5 × 109 CFU/g of S. cerevisiae) resulted in their growth being similar to the group fed a high-density diet. However, Ko and Yang [67] reported that there was no difference in the growth of pigs fed with yeast-containing complex microorganisms compared to the control group. The reason for the conflicting results could be due to differences in the combination of different probiotics [23], but could also be explained by differences in growth phase of the animals [68]. According to results of Giang [69], Giang et al. [70], the effect of probiotics decreased with increasing age.
Processing methods, as well as combinations of complex probiotic strains, may have different efficacy in pig growth, as their success in providing beneficial effects to the host depends on their ability to withstand thermal, osmotic, and oxygen stressors during processing and storage. In addition, Ross et al. [71] reported that the strain’s vitality under thermal and oxygen stressors during processing and storage determines its potency. In a study by Choi et al. [60] differences in growth occurred in pigs fed the same combination of probiotics produced at different drying temperatures. As such, the efficacy for pig growth may vary depending on the processing method. To and Etzel [72] reported that general freeze-drying or spray-drying caused microbial cell damage and death, reducing efficacy and Choi et al. [61] reported that the solid fermentation method had better performance and nutrient retention of probiotics than liquid fermentation. In addition, Liu et al. [18] reported that even if the same complex probiotics were supplemented, there was a difference in efficacy depending on the feed fed.
In addition to growth promoting properties, it has been well documented that probiotics also exert positive effect on nutrient digestibility. For instance, previous studies proved that dietary complex probiotics supplementation could enhance the apparent total tract digestibility (ATTD) of dry matter and gross energy in weaning pigs [60,73]. Another study reported that dietary complex probiotics (L. acidophilus 4.0 × 108 CFU/g, B. subtilis 4.8 × 109 CFU/g and S. cerevisiae 1.0 × 107 CFU/g) supplementation stimulated the ATTD of nitrogen in weaning pigs [60]. When Hu et al. [74] evaluated the digestibility of energy, crude protein, calcium, and phosphorus in the complex probiotics (L. fermentum, S. cerevisiae and B. subtilis) fed group compared to the control group during the entire growth period of pigs from weaning to finishing, it was confirmed that protein digestibility was improved only during the fattening period. Regarding these results, Kim et al. [73] described that the digestive system of young pigs was insufficiently developed, the enzyme secretion activity was low, and the microflora was unstable compared to adult pigs, so the digestibility improvement effect through probiotic feeding was shown only in young pigs. As such, enzyme production by probiotic microorganisms contributes to improved nutrient ATTD in pigs due to probiotic treatment. Aguilar et al. [75] reported higher amounts of certain enzymes or organic acids produced from solid fermentation than those obtained from liquid fermentation. Undigested protein weakens the intestinal wall and is a major cause of diarrhea, but protein hydrolyzed by microbial enzymes into free amino acids and smaller soluble forms is easier to digest than insoluble protein [76]. According to Tonheim et al. [77], solid fermentation of complex lactic acid bacteria increased water-soluble protein compared to liquid fermentation, and it was observed that there was a difference in protein digestibility depending on the processing method.
Maintaining a healthy gut is important for pigs to efficiently digest and absorb dietary nutrients. The gut microbiome, an essential component of a healthy gut, forms a complex ecosystem and plays a crucial role in preventing diarrhea and disease by developing appropriate intestinal architecture and an effective immune system in a symbiotic relationship with the host [2,78,79]. Disruption of the gut microbiome may increase the risk of diarrhea. Probiotics are known to help balance the gut of the host by creating microbiological conditions in the gut, suppressing harmful microbes and favoring beneficial microbes [80]. Probiotics can secrete organic acids, such as lactic acid and acetic acid, which lower the gut pH and competitively exclude pathogenic bacteria through high affinity for nutrients or attachment sites in the intestine [81]. This creates a favorable environment for the native gut microbial community and reduces the possibility of pathogenic colonization [58]. Additionally, some probiotics, like S. cerevisiae, can metabolize or assist in the detoxification of specific inhibitory compounds, such as amines or nitrates, and remove essential oxygen from the anaerobic gut ecosystem [82]. These mechanisms explain the effects of probiotics in regulating the gut microbial balance.
In pigs fed probiotics, the Lactobacillus/Coliform ratio, commonly considered an indicator of gut health, is improved [83,84]. Choi et al. [61] reported that a complex probiotics preparation (L. acidophilus 4.0 × 108 CFU/g, B. subtilis 4.8 × 109 CFU/g and S. cerevisiae 1.0 × 107 CFU/g) was effective in reducing the coliform and Clostridium and improving the Lactobacillus spp. population in the ileum and Bifidobacterium spp. population in the cecum of weaning pigs. Choi et al. [62] similarly reported that dietary multi-species probiotics (L. acidophilus 4.0 × 108 CFU/g, B. subtilis 4.8 × 109 CFU/g and S. cerevisiae 1.0 × 104 CFU/g) improved cecal Lactobacillus spp. populations, but reduced the cecal E. coli counts in weaning pigs (d 28). Kim et al. [85] also found higher levels of Lactobacillus spp. in the ileum of pigs fed a diet supplemented with complex probiotics.
Czech et al. [86] reported that the combined use of S. cerevisiae with probiotics showed a significantly greater impact on reducing the total colonic bacteria compared to using typical probiotics alone. This is because S. cerevisiae can produce antibacterial substances and exerts antagonistic effects against several bacterial pathogens, including E. coli [41,42]. Additionally, the complex structure of the cell wall of S. cerevisiae and mannan bind to toxins released by pathogenic bacteria, detoxifying them and excreting them out of the body [87,88]. The production of antibacterial substances and the structural characteristics of S. cerevisiae play an important role in reducing the number of pathogens in the intestinal tract and creating an environment in which beneficial bacteria can dominate [89,90]. The biodiversity and stability of the gut microbial ecosystem not only benefits animal nutrient utilization but also animal health and productivity performance [91,92].
Reducing the number of pathogenic bacteria in the gut can improve the proliferation of epithelial cells to form villi, thus improving intestinal morphology [93]. The villus height and crypt depth, which are the structures of the intestinal mucosa, serve as indirect indicators of intestinal health, specifically reflecting the maturity of intestinal development and functional capacity [11,60,85]. Longer villi are associated with increased nutrient absorption and utilization [31,94]. In the studies by Choi et al. [60,61] weanling piglets fed a complex of L. acidophilus, B. subtilis, and S. cerevisiae showed no difference in villous structure. However, in the study by Choi et al. [62], villus height and crypt depth increased in the duodenum, jejunum, and ileum.
Pan et al. [93] administered a conjugated probiotic to ETEC K88 challenged pigs and evaluated its efficacy on intestinal morphology and permeability. Pigs challenged with ETEC K88 showed severe villus atrophy and a decrease in the villus height to crypt depth ratio. However, feeding complex probiotics to pigs challenged with ETEC K88 mitigated the negative effects of the challenge on intestinal morphology, and there were no significant differences compared to the non-challenged and antibiotic-treated groups. ETEC colonizes the small intestine and releases enterotoxins, impairing intestinal barrier function and increasing intestinal permeability. This can indirectly lead to fluid loss and facilitate the invasion of pathogenic bacteria [95,96]. When the intestinal barrier is damaged, the permeability of toxins such as endotoxins and lipopolysaccharides from Gram-negative bacterial cell walls into the bloodstream increases [97]. Additionally, the serum diamine oxidase levels also increase [98]. Pigs challenged with ECEC and supplemented with complex probiotics showed significantly lower levels of serum diamine oxidase and endotoxin compared to the control group (p < 0.05). Additionally, there was a significant increase (p < 0.05) in the amount of occludin protein, a component of tight junctions, indicating that complex probiotics improved intestinal permeability and enhanced tight junction integrity. These positive changes in small intestine morphology contribute to improved intestinal health and performance, greater ability to absorb nutrients, and prevention of diarrhea [99–101].
In addition to its function in nutrient digestion and absorption, the GIT of pigs also plays a crucial role in maintaining immune homeostasis. The gut is considered the largest immune organ in the body, accounting for over 70% of the body’s immune cells [102–104].
Previous studies have reported positive immunomodulatory effects of complex probiotics containing S. cerevisiae when fed to pigs. In the research by Czech et al. [86], the use of S. cerevisiae or a combination probiotic containing S. cerevisiae led to a decrease in the number of neutrophil granulocytes and eosinophils, while increasing the number of lymphocytes and immunoglobulin G (IgG) levels. In the study by Phaengphairee et al. [105], the efficacy of complex probiotics containing S. cerevisiae on immune and antioxidant stress markers in weaning pigs was evaluated, and similar results were observed. Pigs supplemented with complex probiotics containing S. cerevisiae showed a decrease in the pro-inflammatory cytokine tumor necrosis factor-alpha compared to the control group. Additionally, the levels of IgG and antioxidant enzymes, such as superoxide dismutase and glutathione peroxidase, increased in the probiotic-supplemented group compared to the control group. However, there were no significant differences in IgM, interleukin-6, and total antioxidant capacity compared to the control group.
Pan et al. [93] reported that feeding conjugated probiotics to ETEC K88-infected pigs increased ileal and jejunal secretory IgA (sIgA) secretion. The sIgA serves as a local immune system in the intestinal mucosal barrier, acting as a primary defense mechanism to limit the access of intestinal antigens to the bloodstream and control intestinal microorganisms [106,107]. These findings suggest that complex probiotics improve gut mucosal resistance against ETEC infection. Thus, an activated defense system can effectively block infections and invasions by pathogens.
When pigs are supplemented with complex probiotics, there have been reports of additional benefits beyond productivity and intestinal health. In a study by Ko and Yang [67], reported that feeding pigs with green tea probiotics containing L. acidophilus 3.2×108 CFU/g, L. plantarum 2.2×108 CFU/g, B. subtilis 4.5×109 CFU/g and S. cerevisiae 5.2×108 CFU/g reported in a significant reduction of thiobarbituric acid reactive substance (TBARS) value in loin meat. Liu et al. [18] suggested that the beneficial effects of the observed complex probiotics on meat quality in the above study are likely due to the probiotics’ antioxidant properties.
Kim et al. [108] reported that supplementation of finishing pigs’ diets with complex probiotics containing S. cerevisiae resulted in reduced drip loss and increased meat redness (a*). Similarly, Liu et al. [109] found that supplementation of probiotics reduced drip loss and cooking loss in pork, but had no significant effect on meat color, pH, and shear force. However, in contrast, Rybarczyk et al. [110] reported that pigs supplemented with probiotics showed lower pH (24 hours post-mortem), increased drip and cooking loss, decreased juiciness and tenderness of the longissimus lumborum muscle, and increased shear force. Further investigation is needed to confirm the efficacy of complex probiotics containing yeast on inconsistent meat quality and to elucidate the underlying mechanisms.
CONCLUSION
In conclusion, the data from previous studies indicate that the use of S. cerevisiae complex probiotics can enhance the productivity in pigs. In general, supplementing swine diets with probiotics has given more positive and consistent effects in weaned piglets than in growing or finishing pigs, which may be due to their inducing better digestibility of feed, improved immunity, and increased resistance to intestinal disorders than young pigs.
The beneficial effects of S. cerevisiae complex probiotics are associated with various mechanisms, including immune modulation, competitive exclusion of pathogenic bacteria, toxin adsorption, and regulation of the gut microbiota. However, the effectiveness of complex probiotics may not always be consistent, as it can be influenced by factors such as the composition of mixed strains, dosage, feed formulation, and the age and health status of the animals. Therefore, more research is needed to explore the efficacy of complex probiotics in pigs.