INTRODUCTION
Extracellular vesicles (EVs), which are released from most cells into the extracellular space, are present in almost all biological fluids [1]. EVs are classified into three categories, namely exosomes, microvesicles, and apoptotic bodies, based on their size, biogenesis, and mechanism of cellular release. Exosomes typically exhibit diameters in the range of 30–150 nm, whereas microvesicles and apoptotic bodies are characterized by particle sizes within the ranges of 100–1,000 nm and >1,000 nm, respectively [2,3]. Exosomes originate from multivesicular bodies that encapsulate a variety of molecules which reflect the cellular environment and subsequently fuse with the cell membrane for release. In contrast, microvesicles and apoptotic bodies are formed directly through the outward budding of the plasma membrane [4]. These exosomes carry molecules, such as RNA, lipids, and proteins, from their parental cells [5].
Circulating exosomes in biological fluids have received extensive attention as noninvasive biomarkers for early diagnosis due to their intercellular communication roles in physiological and pathological processes [6–8]. Although many comparative studies have been conducted on exosome isolation methods from biological fluids, a universally-standardized isolation method does not exist. The advantages and disadvantages of the most commonly-used exosome isolation methods have been previously reported [9–11], and the selection of an exosome isolation approach relies on the source matrix complexity or downstream analysis [12,13]. Blood is a compelling source for exosome clinical application due to the non-invasive sample collection technique and the potential for performing retrospective studies through bio-banking [14]. However, isolating exosomes from serum or plasma is particularly difficult owing to the presence of serum proteins such as albumin, globulin, and lipoproteins, which have a similar size range to exosomes [15,16]. Hence, it is imperative to consider potential variances in the composition of plasma and serum among diverse species, as this strongly influences enhancing the purity of exosome isolation methodologies [17–19]. This implies that the optimal method for isolating exosomes from bovine serum may differ from the method used in human serum. Research on the utilization of exosomes in cattle is limited [20–21], and the studies on exosome isolation have focused on milk and plasma samples [22,23]. Nevertheless, exosome research is crucial in the field of production animal diseases due to its potential to significantly contribute to economic benefits through the early diagnosis of chronic infectious diseases that are difficult to diagnose and provide an in-depth understanding of disease mechanisms.
In exosome research, one of the most critical factors is establishing the most optimal and efficient method for isolating EVs. Ultracentrifugation (UC) is the most widely-used technology for separating exosomes, with approximately half of the researchers opting for this method [9]. Despite being suitable for large-capacity sample processing, UC has the disadvantage of generating many impurities, including non-EV particles such as lipoproteins and protein aggregates. Recently, diverse kits have been commercialized for the rapid and convenient isolation of EVs from serum [16,24]. These include the size exclusion chromatography (SEC) method and membrane affinity separation method [11]. SEC using qEV columns (Izon Science, Addington, New Zealand) allows the separation of EVs larger than 70 nm from plasma proteins. However, SEC has limitations including the relatively-low vesicle yield due to multiple fractions which requires additional pooling and concentration steps [25]. ExoEasy kit ([EE], Qiagen, Hilden, Germany), which was initially evaluated by Enderle et al., uses a membrane affinity spin column based on universal biochemical feature specifications for exosomes [25,26]. Therefore, we chose commonly used exosome isolation methods, including UC and commercial kits such as qEV and EE. Additionally, to ensure an equal amount of serum sample, we concentrated the samples using the UC method before applying the SEC (qEV) method. Similarly, in other studies, combinations of two or more methods have been proposed to overcome the limitations of single-method exosome isolation. Results from isolating exosomes from human plasma using a combination of UC and SEC detected a more diverse range of proteins than using UC alone [27]. Likewise, for bovine plasma exosomes, a combination of UC and SEC was reported to yield a higher exosome yield compared to SEC alone [23].
This study aimed to compare the efficiency and purity of UC, ultracentrifugation SEC (US), and EE methods of exosome isolation from bovine serum. We evaluated the yield, size distribution, and purity of the isolated exosomes, as well as their RNA size range.
MATERIALS AND METHODS
Animal management and sample collection were performed in accordance with the Animal Ethics Committee of the National Institute of Animal Science, Republic of Korea (approval no. NIAS 2022-0559). For exosome isolation, blood was collected from three clinically healthy Holstein cows and a total of 60 mL of blood was collected from the jugular vein using 20 mL syringe equipped with a 18G needle. Blood was collected in serum separator tubes (BD Vacutainer, Franklin Lakes, NJ, USA) and placed upright for 30 min to allow for the red blood cell clot formation. This was followed by centrifugation at 3,000×g for 15 min at 4°C. The supernatants of individual serum samples were pooled and diluted at a 1:1 ratio with Dulbecco’s phosphate-buffered saline ([DPBS], Gibco, Grand Island, NY, USA) to decrease their viscosity. Differential centrifugation steps were conducted to remove cellular debris at 300×g for 10 min and then 12,000×g for 30 min at 4°C [11]. The supernatant was finally filtered through a 0.22-μm filter (Corning Costar, Burlington, MA, USA).
Exosomes were extracted from 10 mL of pre-treated bovine serum using three different methods: UC, US, and EE (Fig. 1). The data are presented as the mean of three independent experimental replicates and each serum volume was isolated three times per replicate. UC was performed according to the protocol reported by Helwa et al. [24] with some modifications. Pre-treated serum was centrifuged at 110,000×g for 70 min at 4°C (Type 55.1 Ti, fixed angle ultracentrifuge rotor, Beckman, Brea, CA, USA, ) to precipitate exosomes. The pellet was reconstituted in DPBS to a final volume of 500 μL and stored at −80°C until further analyses. UC method was performed twice: with and without the SEC. For the US method, 500 μL of exosome pellets obtained by UC were divided into13 fractions using the qEV column (Izon Science, Addington, New Zealand). To confirm the exosome-containing fraction of the SEC, the protein expression of TSG101 and CD81 was investigated in the whole fraction (fractions 1-13) An equal amount (30 μg) of protein was tested by Coomassie blue stain (Fig. 2A) and for anti-TSG10 and CD81 by western blot. As a result, the expression of TSG101 was confirmed in the F1 to F4 fractions, and the expression of CD81 was confirmed in the F1 and F2 fractions (Fig. 2B). Finally, fractions F1 and F2, which expressed both TSG101 and CD81, were identified as enriched in exosomes, pooled and utilized as US particles in the study. The same volume (10 mL) of pre-treated serum was applied to EE methods (Qiagen, Hilden, Germany) according to the manufacturer’s instructions [26]. Exosomes was captured and washed using the reagents provided in the kit. The maximum serum-based sample volume processed per column was fixed at 4 mL, resulting in the use of three columns. Exosomes were eluted using 400 μL of elution buffer per column, and then concentrated by UC before being suspended in 500 μL of PBS.
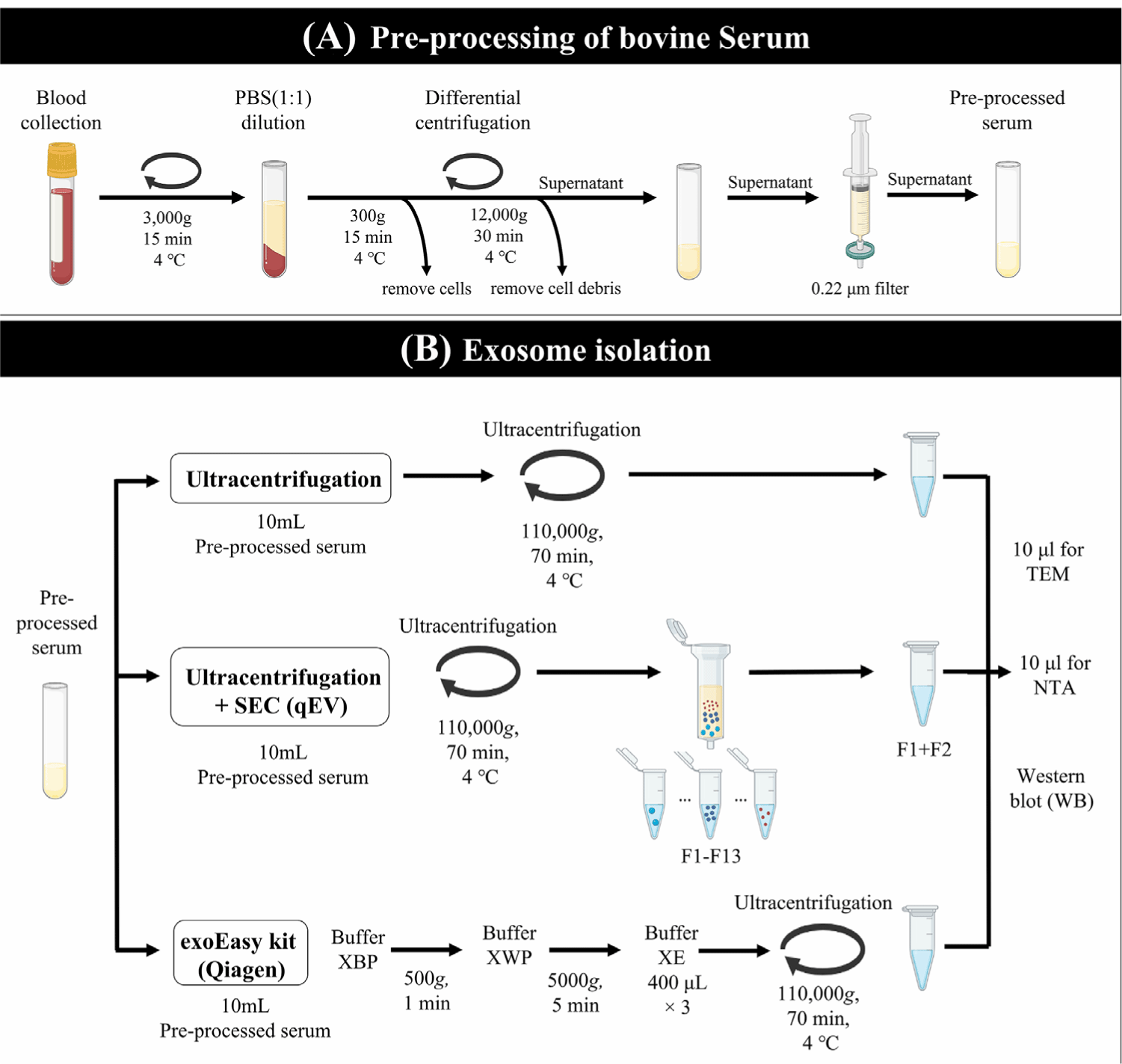
Copper grids were glow-discharged to remove adsorbed hydrocarbons, rendering them hydrophilic. A total of 5 μL of enriched exosomes were added onto formvar-coated copper grids for 2 min, then washed in ultrapure water and negatively stained with 1% uranyl acetate. The samples were then visualized using HT7800 TEM operated at 80 kV, and images were captured using an Olympus Soft Imaging Veleta digital camera.
The UC and EE samples were diluted 50- and 6-fold, respectively, and the US was measured using the original, and the original concentration was calculated considering the dilution factor. NTA measurements were performed using the PMX220 TWIN instrument. The manufacturer’s default software settings for the particles were selected accordingly. For each measurement, two cycles were performed by scanning 11 cell positions and capturing 270 frames per position using the following settings: autofocus; camera sensitivity for all samples, 80.0; shutter, 100; and cell temperature, sensed. After capturing, the videos were analyzed using the software ZetaView version 8.05.16, with the following specific analysis parameters: maximum area, 1,000; minimum area, 10; minimum particle brightness, 30; hardware, embedded laser: 40 mW at 488 nm.
Protein samples were prepared by adding 10 μL of 10x radioimmunoprecipitation assay buffer with a protease inhibitor cocktail (Genedepot, Baker, USA) to 90 μL exosome samples suspended in PBS. The samples were mixed and lysed on ice for 15 min. The total protein content of exosomes was measured using a Pierce Micro BCA Protein Assay Kit (Sigma-Aldrich, Missouri, USA). Afterwards, 4x Laemmli buffer (25 μl, Bolt LDS sample buffer, Life Technologies, CA, USA) and 10x reducing agent (10 μl, Bolt antioxidant, Life Technologies, CA, USA) were added, and the samples were vortexed and incubated for 10 min at 70°C. Protein samples (30 μg) were loaded and separated using Bolt Novex 4–12% Bis-Tris Gels (Life Technologies). The proteins were transferred onto a polyvinylidene fluoride membrane (Life Technologies) using a mini-blot module system. Membranes were blocked for 1 h in 5% bovine serum albumin blocking solution and incubated overnight with the primary antibodies anti-TSG101 (catalog #ab125011), anti-CD81 (catalog #NBP1-77039) at 4°C, anti-HSP70 (catalog #EXOAB-Hsp70A-1), and anti-albumin (catalog #A11133), followed by the secondary antibody anti-rabbit IgG (catalog #ab205718) or anti-mouse IgG (catalog #ab6728). Targeted proteins were visualized using West-Q pico ECL solution (Genedepot, Baker, TX, USA) on X-ray films. Another gel was prepared in the same manner and stained with coomassie blue.
Total RNA was isolated using the miRCURY RNA isolation kit (catalog #300110) following the manufacturer’s instructions. Briefly, up to 50 μL suspended exosomes were processed with RNA isolation columns and buffers provided by the manufacturer. A final volume of 50 μL RNA solution was collected from each sample using the supplied elution buffer. The RNA size range were analyzed on an Agilent 2100 bioanalyzer using an RNA 6000 Pico Chip (Agilent Technologies, Santa Clara, CA, USA).
Data were analyzed using GraphPad Prism software (version 10.0.2, La Jolla, CA, USA). One-way ANOVA followed by post-hoc Tukey’s test were conducted. A p-value less than 0.05 was considered as statistically significant; p-values of *<0.05, **<0.01, and ***<0.001. Data are presented as mean ± SEM.
RESULTS
Exosomes were isolated from the pre-treated bovine serum using three methods: UC, US, and EE. In preliminary trials, out of 13 fractions obtained using the US method, exosome-specific markers TSG101 and CD81 were found to be co-expressed exclusively in F1-2, not in other fractions. Consequently, F1-2 fractions were pooled for all subsequent US method (Fig. 2B). TEM analysis of exosomes obtained from all isolation methods revealed round or cup-shaped particles, a typical exosome morphology (Fig. 3A). However, torn or broken exosomes were also frequently observed in UC, whereas intact exosomes were more commonly observed in the US method. In serum exosome fractions prepared using the EE kit, exosome-like structures were observed, but their occurrence was much less frequent than those in the US eluates.
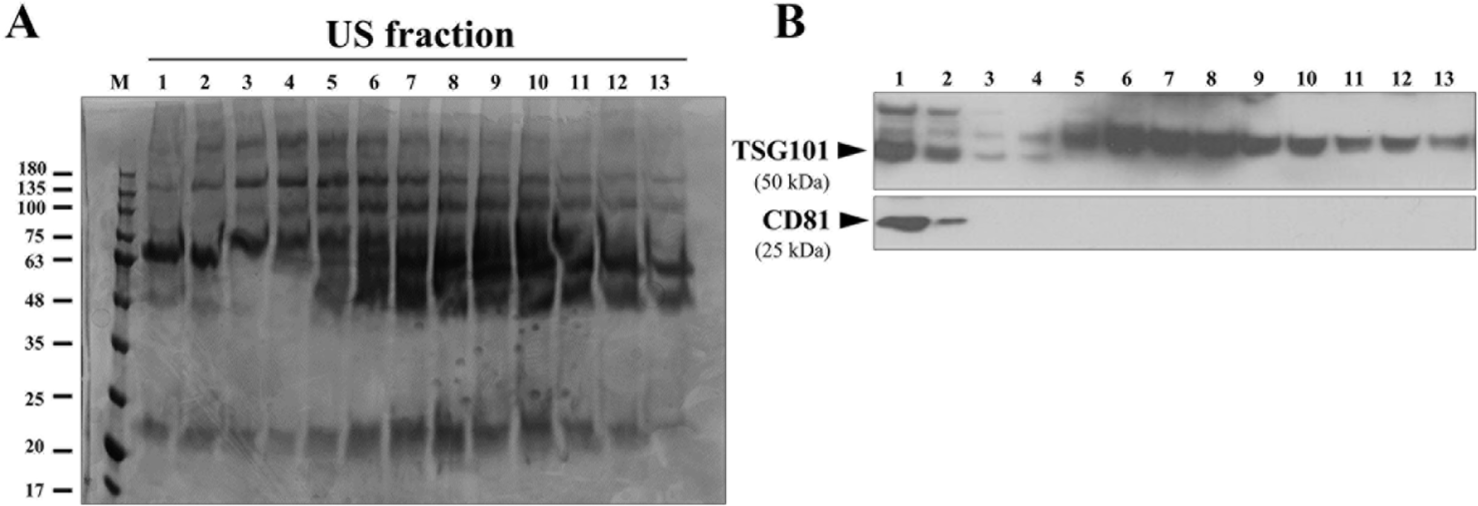
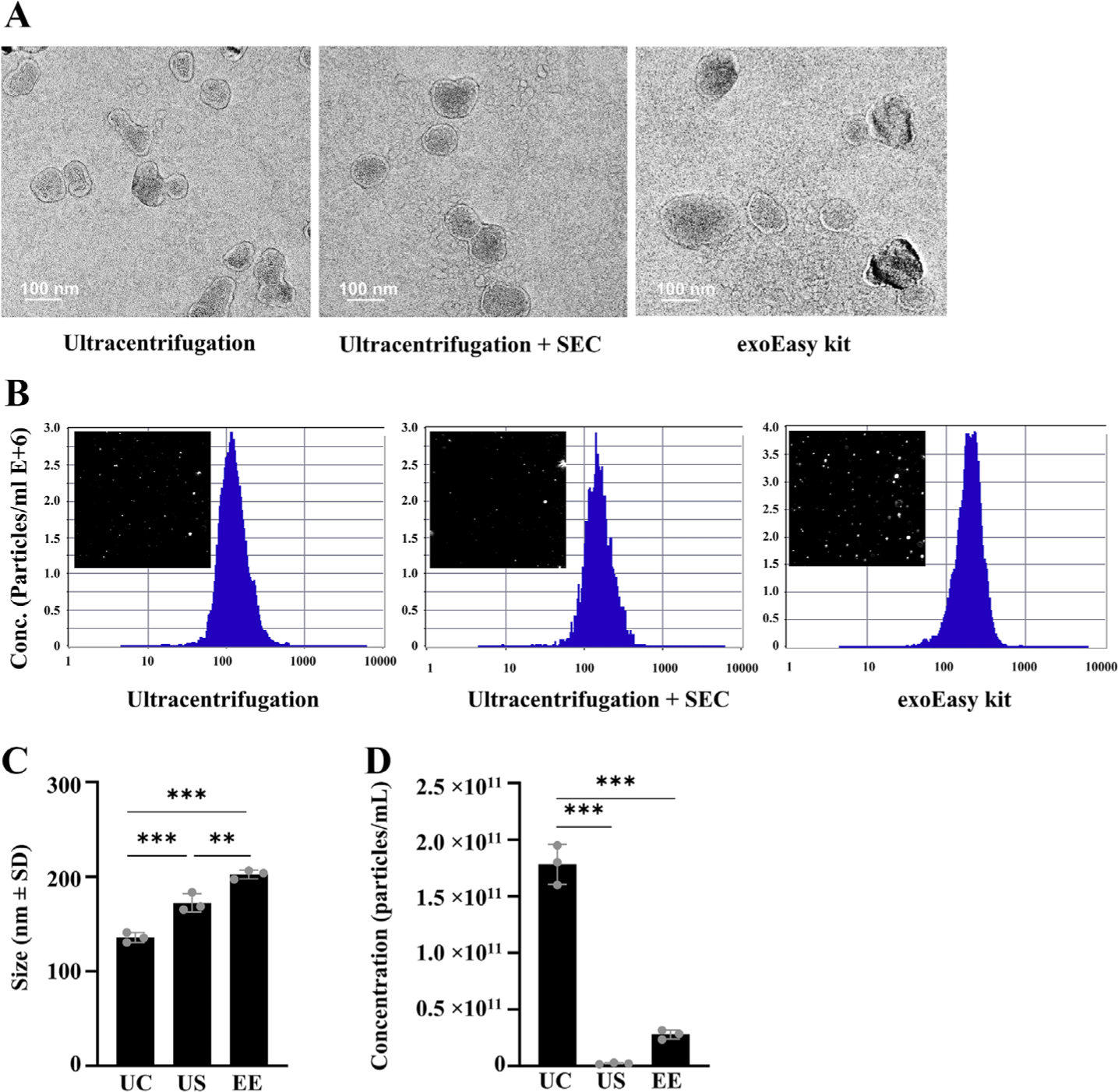
Comparative analysis of the size distribution and total number of exosomes was conducted using NTA. Differences in the size of exosomes were observed along with morphological differences of exosomes depending on the isolation method. Particles isolated from UC (median diameter ± SD, 136.2 ± 0.43 nm) were within the size range of exosomes (50–150 nm). In contrast, particles isolated using the EE (202.3 ± 1.86 nm) and US (172.4 ± 4.6 nm) methods exhibited a broader size distribution (Fig. 3B). The relatively larger particle diameter obtained with the EE method are consistent with the findings of the TEM analysis, confirming the significant heterogeneity of particles isolated using the affinity spin column. Analysis of the particle concentration using NTA showed that the UC method yielded the highest concentration of exosomes particles (1.78 × 1011 particles/mL) followed by the EE (2.78 × 1010 particles/mL) and US (2.28 × 109 particles/mL) methods (Fig. 3C).
Purity was assessed by measuring the protein content and expression of exosome-enriched proteins of EVs isolated from bovine serum using three methods. The UC pellets had significantly higher (p < 0.01 and p < 0.001) total protein concentrations compared to those of US and EE, with approximately 115- and 2.3-fold differences, respectively (Fig. 4A) (mean ± SD; UC, 1,029 ± 122 μg/mL; US, 9.2 ± 1.2 μg/mL; EE, 428 ± 34 μg/mL). Subsequently, we conducted western blot analysis using equal protein loading, enabling the direct assessment of exosome sample purity by comparing the enrichment of proteins recognized as exosomal markers with the presence of contaminating serum proteins (Fig. 4B). TSG101 was detected in exosomes isolated by all methods, whereas CD81 was only detected in exosomes enriched by the US method and not in those isolated by the UC and EE methods. Similar results were observed for HSP70, with weak signals detected using the US method and undetectable levels in exosomes using the UC and EE methods. Albumin was present in the UC and EE particles but not in those obtained using the US method. Correspondingly, as a result of coomassie blue staining of the total exosomal protein showed a distinct band in the 63–75 kDa range for UC, suggesting albumin contamination (Fig. 4C). Exosome purity was estimated by calculating the ratio of the particle count to protein concentration for evaluating the extent of contamination with non-EV proteins. Higher particle-to-protein (p:p) ratio values indicate exosome enrichment in the US samples (mean± SD; US, 2.83 ± 0.10 × 108 particles/μg; UC, 1.6 ± 0.14 × 108 particles/μg; EE 0.66 ± 0.07 × 108 particles/μg; Fig. 4D). The lower p:p ratio observed using the UC and EE method was likely due to the presence of co-separated proteins, which is in line with the protein concentration results.
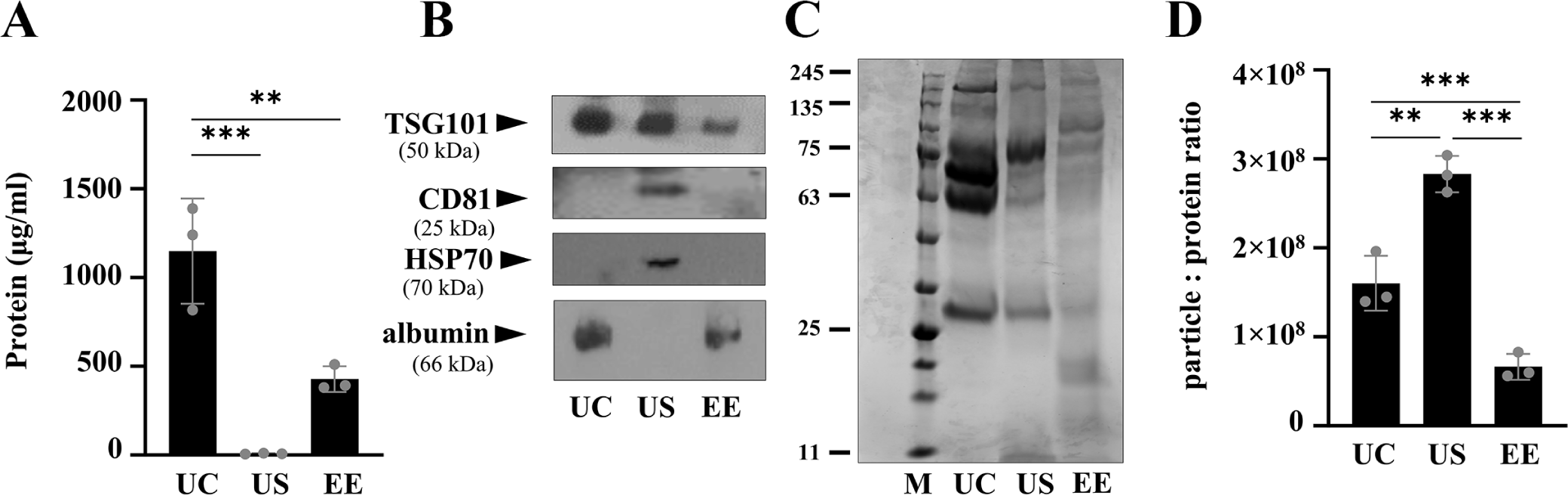
Exosomes are an important source of RNA-based biomarkers; thus, the RNA profile of exosomes from UC, US, and EE methods were evaluated using a bioanalyzer. According to the electropherogram and gel images from the Bioanalyzer, peaks and bands were observed at the location of 18S rRNA (1,900 nt) in the RNA extracted from separated serum [28]. However, the RNA extracted from EVs isolated using the UC, US, and EE methods did not display 18S (1,900 nt) or 28S rRNA (4,700 nt) bands (Figs. 5A and 5B). Furthermore, the band corresponding to small RNA (25-200 nt) was indistinct in UC method, whereas in the EE method show the most well-defined band. Lastly, US showed a faint but distinguishable single band in the small RNA size range (Fig. 5A).
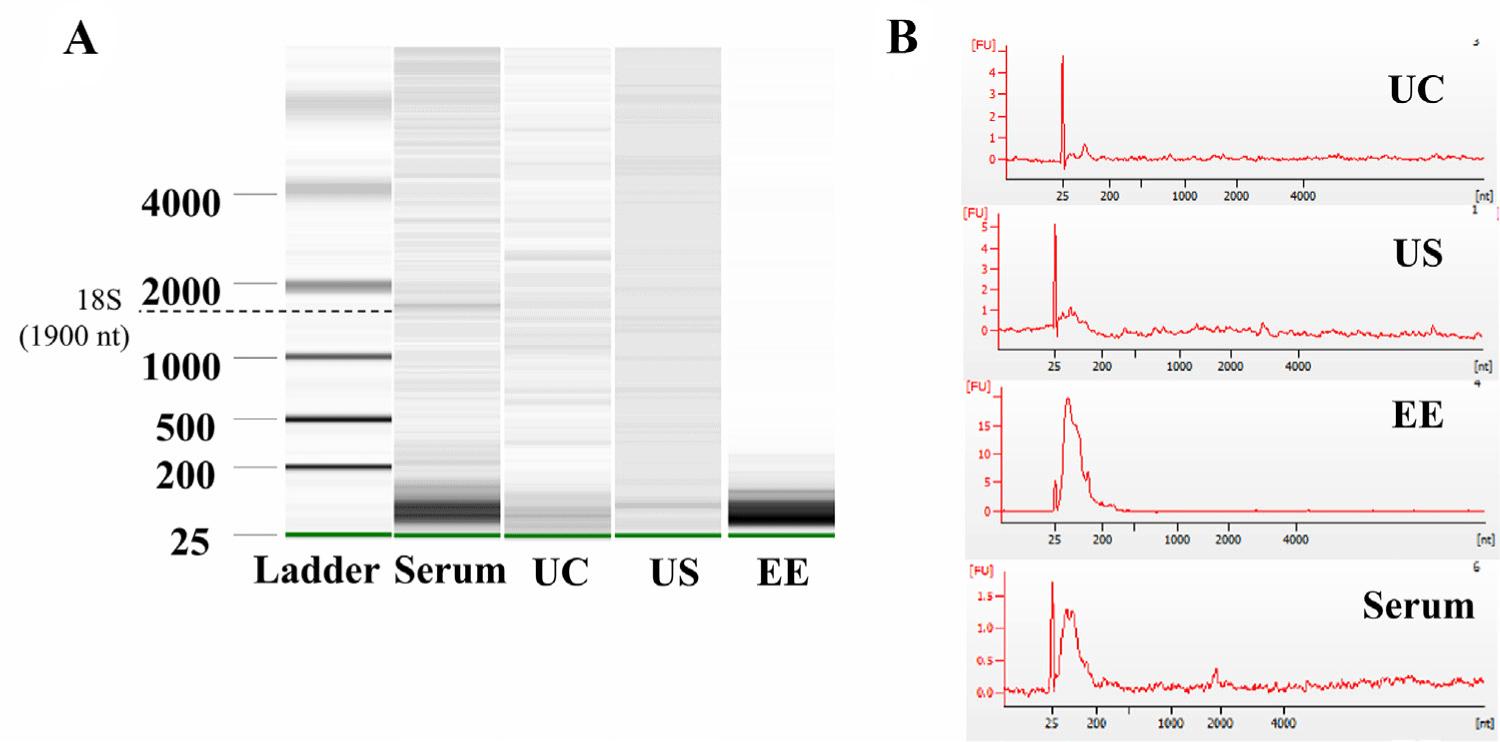
DISCUSSION
In veterinary medicine, bovine exosomes exhibit significant potential for diverse diagnostic research and biomarker discovery. Particularly, the development of an optimal method for obtaining highly-pure exosomes from bovine serum would be beneficial for further clinical applications. Isolation of pure exosomes from serum is technically challenging due to its high viscosity and complex composition including lipoproteins, ribonucleoproteins, and other types of vesicles. In human exosome research, several studies have already been conducted to efficiently isolate high-pure exosomes from serum. However, translation into bovine serum poses distinct challenges that remain unexplored. To our knowledge, only one comparative study has reported efficient exosome isolation from bovine plasma [23]. To date, there has been no report on research on efficiently isolating exosomes from bovine serum, and research on bovine serum exosomes has focused on removing exosomes derived from fetal bovine serum due to their effect on cell differentiation or proliferation [29]. The previous studies only focused on removing exosomes, however, for diagnostic purposes, isolating intact exosomes is important, differentiating the present study from previous ones. In this study, we compared three isolation techniques: UC, US, and EE. Additionally, to test whether the exosome isolation method used in humans is also suitable for bovine serum, we analyzed the characteristics and identities of particles obtained by different methods.
TEM analyses showed that the UC method produced particles with damaged membrane. This may be due to the high centrifugation speed and repeated washing steps, which could potentially compromise the integrity of the exosome membranes. When SEC was employed following UC, the characteristic double membrane and rounded morphology of the exosomes were well maintained, indicating the potential exclusion of damaged exosomes during the SEC process. The average diameter of the US isolation method particles was 172 nm; this exceeded the commonly accepted size range of 30–150 nm in the field of exosome research. However, according to the definition of exosomes presented in the recent MISEV2023 guideline, exosomes are demonstrated to be subtypes of small EVs and the diameter of endosomes’ lumenal vesicles is typically a particle smaller than 200 nm [30]. Recent studies have highlighted the significant influence of isolation techniques on the observed heterogeneity and size distribution of exosomes. Notably, research employing SEC has demonstrated the capability of isolating exosomes from plasma with sizes up to 200 nm [31]. This finding suggests a potential need to reconsider the traditionally accepted upper size limit of 150 nm for exosomes, acknowledging the method-dependent variability in exosome size. Studies have demonstrated that SEC is effective in removing smaller serum-derived contaminants like high-density lipoprotein and albumin, which are co-precipitated during UC [25]. Consequently, the use of SEC post-UC may result in an increased mean diameter of isolated particles, attributed to the selective removal of these smaller contaminants. Thus, although exosome isolation with the SEC may increase the efficiency of the experiment and improve the purity of the results, it is also worth considering that the exclusion of exosomes smaller than 70 nm may omit information about exosomes smaller than that size. Particles obtained by EE method contained irregularly shaped particles with larger diameters resembling protein aggregates [32]. Correspondingly, the NTA findings revealed that the particles from the EE method have large particles with a diameter exceeding the typical size range of exosomes. Similarly, other researchers have identified larger particles with a diameter of 210 nm when using the EE kit. This phenomenon is likely attributable to the ability of the EE method to utilize extensive membrane hydrophilicity [25].
Using NTA analysis, we identified that UC yielded a significantly higher (p < 0.001) number of particles compared to the US and EE methods. Similarly, UC samples had higher (p < 0.001 and p < 0.01) total protein concentrations compared to those of US, and EE respectively. UC has been adopted as the classic techniques for exosome isolation, however, the pellet from high-speed spin could contain protein aggregates, lipoproteins, and other contaminants. The limitations of NTA in differentiating between exosomes and similar-sized non-exosomal particles, such as lipoproteins or protein aggregates, suggest a potential overestimation of exosome concentrations in samples prepared by UC [33]. Additionally, the absence of exosome-specific markers CD81 and HSP70 in UC samples, despite using the same protein amount as the standard, indicates a lower presence of actual exosomes among the serum impurities. This underscores the need for cautious interpretation of particle concentrations and compositions in exosome research.
One of the major sources of protein contaminations in serum exosome preparation is albumin. We confirmed the albumin presence in UC and EE isolates using immunoblotting assay, but it was not detected in the US method. This is consistent with the results of previous exosome proteomics analysis showing that application of SEC after UC is effective in albumin removal [27]. Similarly, Baranyai et al., also reported the contamination of albumin in UC isolates [34]. Exosome protein markers are classified based on their biological and functional properties. The main markers include the tetraspanin family (CD9, CD63, and CD81), endosome-associated proteins (TSG101, ALIX), and heat shock proteins (HSP70, HSP90) [35]. However, even with the same tetraspanin family, it can be used to differentiate the subpopulations of exosomes, and CD9 or CD81 has been studied as a more commonly expressed protein than CD63. In this study, CD81, TSG101, and HSP70 were selected for analysis considering the ubiquitous identification and functional importance of exosomes [36]. In US samples, all three markers (CD81, TSG101, and HSP70) were expressed while only TSG101 was detected in UC and EE samples. The TSG101 and CD81 expression in US samples was consistent with other previous reports. Koh et al. showed TSG101 expression and Wei et al. confirmed CD81 expression in US fractions [23,37]. However, the protein expression data in this report did not entirely align with previous report by Stranska et al., who reported the expression of TSG101 in UC and SEC methods, but lack of exosome marker expression in EE samples [25]. This discrepancy may be due to the differences in the amounts of proteins used for western blot. If the sample contains relatively limited amount of target protein, it may not be detected by blotting. Alternatively, there may be a difference in the characteristics of exosomes that originate from different species (e.g. humans versus cattle). Despite reports indicating that the particle count measured by NTA may not accurately reflect the actual number of exosomes, many studies still estimate the purity of exosomes by calculating the ratio of the particle count to protein concentration. The UC and EE methods resulted in a lower particle-protein ratio compared to the US method, indicating the potential co-isolation of proteins with UC and EE. However, the US method, demonstrating a high particle-protein ratio along with identifiable exosome markers (TSG101, CD81, and HSP70), suggests a superior purity of exosome isolation, free from albumin. This underscores the advantage of integrating UC and SEC techniques for achieving enhanced exosome purity in preparations. Similarly, studies on the separation of human plasma exosomes have shown that combining UC and SEC significantly improves the separation and purity of exosome proteins in proteomic analysis using liquid chromatography-mass spectrometry (LC-MS) [27]. In future studies utilizing the US method from bovine serum, it will be essential to conduct comprehensive analyses of both miRNA and protein profiles.
In order to discover biomarkers through analysis of miRNA in exosomes, it is essential to check the content of miRNA by analyzing the profile of small RNA in exosomes. Although the higher purity of EVs extracted from US compared to EE and UC, bands of small RNA (25–200 nt) were most prominent in EE samples, followed by US, which showed a single distinct band. Tang et al. observed that extracting exosomes from serum through commercial kits (exoQuick and Total Exosomes Isolation Reagent) resulted in a higher amount of exosomal RNA than UC [28]. However, the method of extracting exosomal RNA was different according to the exosome extraction method, UC condition details were not described in the methods, making accurate comparison difficult. However, it can be inferred that there is a change in miRNA extraction yield according to the exosome extraction method. While our study concentrated on miRNA profiling and did not delve into the broader functional aspects of exosomes, including cytokines and proteins, this marks a limitation. Future research will not only expand to include a comprehensive analysis of exosomal proteins and cytokines but will also aim to distinguish between exosomal profiles in normal versus pathological conditions, shedding light on their potential diagnostic and therapeutic implications in various diseases.
In conclusion, combining UC and SEC is suitable for separating bovine exosomes with high purity despite low particle number. However, considering that EE has the highest miRNA content, it suggests the need to carefully select the exosome isolation method depending on the purpose of exosome analysis. This study significantly contributes to the advancement of the field of exosome biomarkers in veterinary medicine and highlights the importance of thorough evaluation of new exosome isolation technologies.