INTRODUCTION
Somatic cell nuclear transfer (SCNT) is a promising technology with potential applications in basic life science research, agriculture and regenerative medicine. The production of live offspring by SCNT has been achieved in many mammalian species [1], including sheep [2], cow [3], mouse [4], pig [5], horse [6], dog [7], monkey [8] etc. The development of SCNT technology in pigs is producing transgenic pigs with specific genetic modifications of somatic cells [9]. As pigs and humans are similar in their anatomy, physiology and body size, the transgenic pigs are readily considered the primary alternative source for xenotransplantation and human disease model [10,11]. Great progress has been made in cloning techniques, and the first successful cloned pig was achieved by SCNT technology in the year 2000 [5]. However, the efficiency of pig cloning by SCNT is still very low, with a success rate of less than 5% [12–14]. Numerous factors are responsible for poor cloning efficiency, including recipient oocyte quality, cell cycle stage of the donor cells, reconstructed embryo activation methods and culture condition. Of these, suboptimal culture conditions have been suggested as one of the most important factors responsible for the low success rate of SCNT techniques.
Lysophosphatidic acid (LPA) is a small glycerophospholipid (molecular mass: 430–480 Da), has been identified as a key signaling molecule participating in the regulation of reproductive functions in mammals [15,16]. LPA shows growth factor-like and hormone-like functions on various animal cells [17], and it can give rise to various cellular responses, including cell proliferation, survival, and differentiation etc. [18–20]. The beneficial effect of LPA on oocyte maturation and/or development of preimplantation embryos has been evaluated in many species such as golden hamster [21], mice [22], pig [23,24], bovine [25–27] and human [28]. For instance, LPA supplementation of the relevant in vitro maturation medium was shown to improve the nuclear and cytoplasmic maturation of golden hamster oocytes [21] and enhance bovine oocyte maturation and embryo development while decreasing apoptosis in these species [25,29]. In pig, previous studies showed that LPA improved the development and quality of porcine in vitro fertilization (IVF) [24]- and parthenogenetic activation (PA) [23]-derived embryos. Collectively, the above reports suggest that LPA could be an interesting addition to an in vitro embryo culture system. However, whether LPA can enhance the development of porcine SCNT embryos, and its possible mechanisms are still not completely comprehended.
To investigate the possible mechanisms of LPA influence the development and quality of porcine SCNT-derived embryos, we cultured porcine SCNT embryos in the chemically defined polyvinyl alcohol (PVA)-based medium, porcine zygote medium-4 (PZM-4), with or without LPA, and examined the cleavage, blastocyst formation, cell proliferation potential, reactive oxygen species (ROS) formation, mitochondrial membrane potential and apoptosis index, as well as the expression levels of pluripotent markers and genes involved in development and apoptosis.
MATERIALS AND METHODS
All chemicals or reagents used in the present study were purchased from Sigma Chemical (St. Louis, MO, USA) unless otherwise indicated. All animal experiments were approved by the Institutional Animal Care and Use Committee of Chungnam National University.
Ovaries of prepubertal gilts were collected at a local abattoir and transported to the laboratory within 2-3 h in phosphate-buffered saline (PBS) solution supplemented with 75 μg/mL potassium penicillin G and 50 μg/mL streptomycin sulfate. Follicular contents were aspirated from antral follicles (3 to 6 mm in diameter) visible on the ovarian surface using an 18-gauge needle attached to a 10-mL disposable syringe. Porcine cumulus-oocyte complexes (COCs) with homogenous cytoplasm and more than two uniform layers of compact cumulus cells were selected for in vitro maturation (IVM).
For porcine oocyte IVM, groups of 50–60 COCs were placed into each well of a four-well multi dish (Nunc, Roskilde, Denmark) containing 500 μL maturation medium (TCM 199) supplemented with 10% porcine follicular fluid, 3.5 mM D-glucose, 0.57 mM L-cysteine, 0.91 mM sodium pyruvate, 75 μg/mL penicillin, 50 μg/mL streptomycin, 10 ng/mL epidermal growth factor, 10 IU/mL pregnant mare serum gonadotropin and 10 IU/mL human chorionic gonadotropin. The COCs were cultured at 38.5°C in saturated-humidity air containing 5% CO2. After 22 h of maturation incubation, the porcine COCs were transferred to the same maturation medium lacking hormones and incubated for an additional 22 h.
Porcine fetal fibroblasts were isolated from fetuses at day 35 of gestation [30]. Cells were cryopreserved upon reaching confluence. Before SCNT experiments, the cells were thawed and cultured in DMEM (11995-073, Gibco, Waltham, MA, USA) supplemented with 10% fetal bovine serum (16000-044, Gibco), 1% MEM-NEAA (11140-050, Gibco) and 0.1% gentamicin reagent solution (15750-060, Gibco), at 38.5°C in a humid 5% CO2 atmosphere.
For SCNT, denuded oocytes were subjected to enucleation, nuclear transfer, fusion and cytoplast activation procedures. Briefly, porcine oocytes were enucleated by aspirating the first polar body and adjacent cytoplasm using an enucleation needle (ORIGIO, Charlottesville, VA, USA) in enucleation medium. A single donor cell was transferred into the perivitelline space of each enucleated oocyte using the same enucleation pipette. The reconstructed embryos were fused and activated with two 100-μs DC pulses of 1.1 kV/cm, using an Electro Cell Manipulator 2001 (BTX, San Diego, CA, USA) in 0.3 M D-mannitol supplemented with 1.0 mM CaCl2·H2O, 0.1 mM MgCl2·6H2O and 0.5 mM HEPES. The activated embryos were washed with PZM-4 (PVA based) and 8-10 embryos were transferred into 100-μL micro drops of PZM-4, covered with mineral oil, and incubated at 38.5°C in a 5% CO2 atmosphere. The day of nuclear transfer was designated as day 1.
The numbers of inner cell mass (ICM) and trophoblast (TE) cells in blastocysts were determined using dual differential staining. Briefly, the zona pellucida (zp) was removed from blastocysts by incubation in 0.5% pronase solution. The zp-free embryos were washed in PBS containing 0.1% (w/v) PVA (PBS-PVA) and transferred into a 1:5 dilution of rabbit anti-pig whole serum (P3164, Sigma) for 1 h at 38.5°C. After being briefly rinsed with PBS-PVA, the samples were incubated with guinea pig complement (S1639, Sigma) diluted 1:10 with PBS-PVA containing 10 μg/mL propidium iodide (PI) and 10 μg/mL Hoechst 33342, for 1 h in the dark at 38.5°C. After being briefly rinsed in PBS-PVA, the samples were mounted on slides with coverslips and observed under epifluorescence microscopy (BX51, Olympus Tokyo, Japan). Blue (Hoechst 33342) and pink (both Hoechst 33342 and PI) colors were taken as representing ICM and TE cells, respectively.
Porcine SCNT-derived embryos were incubated with 10 μM 2’,7’-dichlorodihydrofluorescein diacetate (H2DCFDA, Invitrogen, Eugene, OR, USA) for 30 min in the dark at 38.5°C. After being briefly rinsed in PBS-PVA, the samples were examined under an epifluorescence microscope (BX51, Olympus). The same instrument parameters were used to normalize the results across the replicates. The fluorescence intensity in each embryo was measured using the ImageJ software (version 1.46r, National Institutes of Health, Bethesda, MD, USA) after background subtraction.
JC-1 dye (T3168, Thermo Fisher Scientific, Eugene, OR, USA) was used to assess the mitochondrial membrane potential. Samples were exposed to 10 μg/mL JC-1 at 38.5°C in the dark for 15 min, washed in PBS-PVA and examined under an epifluorescence microscope (BX51, Olympus). Fluorescence images were captured and saved as graphic files in TIEF format. The ratio of red florescence (J-aggregates, corresponding to activated mitochondria) to green florescence (J-monomers, corresponding to less-activated mitochondria) was used to calculate mitochondrial membrane potential using the ImageJ software after background subtraction.
The porcine SCNT-derived blastocysts were fixed in 4% paraformaldehyde for 30 min, and then permeabilized in 0.5% (v/v) Triton X 100 for 30 min. After permeabilization, the samples were washed with PBS-PVA, blocked with 3% bovine serum albumin (BSA) in PBS for 1 h, washed in PBS containing 0.5% BSA and 0.1% gelatin (PBG) for 20 min and incubated overnight at 4°C with anti-Oct-3/4 (1:200, sc-5279, Santa Cruz Biotechnology, Santa Cruz, CA, USA). The samples were then washed in PBG for 20 min and then reacted with goat anti-mouse IgG-R (1:200, sc-2092, Santa Cruz Biotechnology) in the dark for 1 h. Negative control samples were processed as described above, except that no anti-Oct-3/4 antibody was added. Finally, all samples were mounted on slides using VECTASHIELD mounting medium containing DAPI (Vector Laboratories, Burlingame, CA, USA) and examined with a Zeiss laser-scanning confocal microscope (LSM5 Live, Carl Zeiss, Germany).
For EdU staining, a Click-iT EdU Imaging kit (C10337, Invitrogen) was used according to the provided instructions. Briefly, porcine SCNT-derived blastocysts were incubated in a final concentration of 10 μΜ EdU solution for 5–6 h at 38.5°C. Blastocysts were fixed for 15 min in 4% paraformaldehyde solution. After being briefly rinsed in PBS containing 3% BSA, the samples were permeabilized with 0.5% Triton X-100 for 20 min, washed in PBS containing 3% BSA, and then reacted with a Click-iT reaction cocktail containing Alexa Fluor 488 azide. After EdU labeling, samples were mounted on slides using VECTASHIELD mounting medium containing DAPI and examined under a Zeiss laser-scanning confocal microscope.
Apoptosis in blastocysts were examined using a TUNEL assay kit (TMR Red, Mannheim, Roche, Germany). Porcine SCNT-derived blastocysts were washed in PBS-PVA and fixed in 4% paraformaldehyde for 30 min. The samples were permeabilized with 0.5% Triton X-100 for 30 min, and then reacted with TUNEL reaction solution for 1 h at 38.5°C in the dark. The samples were then washed in PBS-PVA and mounted on slides using VECTASHIELD mounting medium containing DAPI. A negative control test was performed as described above, except that no terminal deoxynucleotidyl transferase was added.
Porcine blastocysts were collected and stored at −70°C until analysis. The expression levels of embryo development-related genes (IGF2R, SLC2A1, DNMT3A, PCNA, CDH1 and DSC2), apoptosis-related genes (BAX, CASP3 and BCL2L1) and pluripotent marker genes (Oct4, Sox2 and Nanog) were analyzed using quantitative real-time PCR. Total RNA was extracted from each sample (10 blastocysts) using an RNeasy Mini Kit (Cat. No. 74104, Qiagen, Valencia, CA, USA), and cDNA was prepared with a TOPscript™ RT DryMIX kit (Enzynomics, Daejeon, Korea) in accordance with the manufacturer’s instructions. Real-time PCR was conducted with a TOPreal™ qPCR 2X PreMIX (SYBR Green with low ROX) kit (Enzynomics) on a CFX96 Touch Real Time PCR Detection System (Bio-Rad, Laboratories, Singapore). PCRs with no template controls were performed for each primer pair. Relative mRNA expression levels were analyzed using the 2−ΔΔCt method. ACTB was used as an internal standard. The sequences of the primers used to amplify each gene are presented in Table 1.
In Experiment 1, we examined the effects of LPA on the development (cleavage and blastocyst formation rates) and embryonic development-related gene expression (IGF2R, SLC2A1, DNMT3A, PCNA, CDH1 and DSC2) of porcine SCNT-derived embryos.
In Experiment 2, we examined the effects of LPA on the cell proliferation potential (the numbers of total, ICM, TE and EdU-positive cells) of porcine SCNT-derived blastocysts.
In Experiment 3, we tested the effects of LPA on ROS formation, mitochondrial membrane potential, apoptosis index and apoptosis-related gene expression (BAX, CASP3 and BCL2L1) in porcine SCNT-derived blastocysts.
In Experiment 4, we tested the effects of LPA on the expression levels of a pluripotency marker protein (Oct4) and pluripotency marker genes (Oct4, Sox2 and Nanog) in porcine SCNT-derived embryos.
Statistical analysis was performed using SPSS 17.0 (SPSS, Chicago, IL, USA). Data were compared using one-way analysis of variance (ANOVA) and t-tests. Percentage data were subjected to arcsine transformation prior to analysis. Each experiment consisted of at least three replicates. The data are presented as the mean ± SEM. A p-value of < 0.05 was considered significantly different.
RESULTS
To determine the appropriate concentration, we pre-tested the influence of different concentrations of LPA on porcine SCNT-derived blastocyst formation rate. We found that 10 μM LPA is the optimal concentration for porcine SCNT-derived embryo development (Fig. 1A). Thus, 10 μM LPA was employed in the subsequent study.
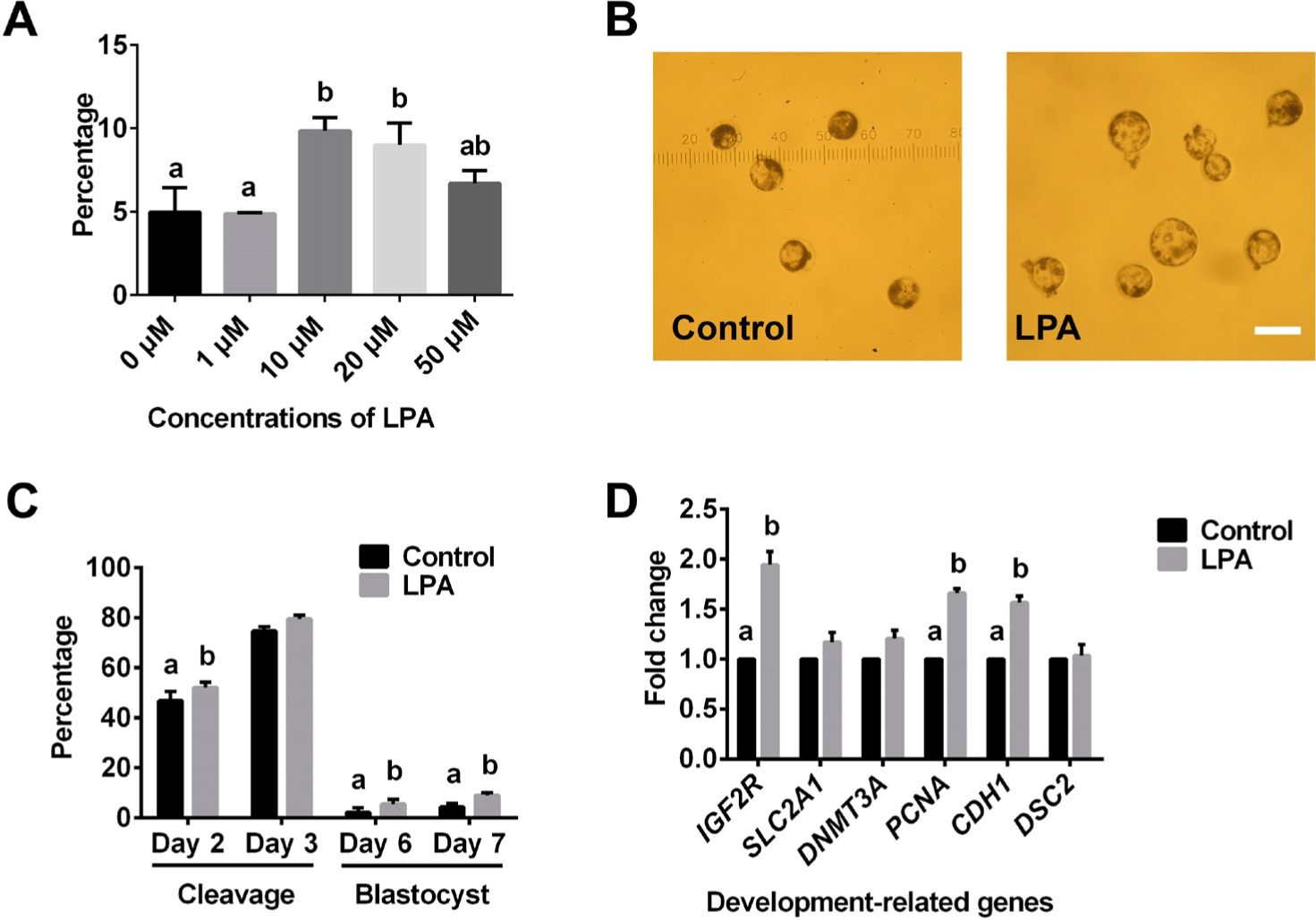
As shown in Fig. 1, LPA significantly increased the cleavage rate at day 2 of culture and increased the blastocyst formation rates at days 6 and 7 of culture (Figs. 1B and 1C). LPA also increased the cleavage rate at day 3 when compared to that of the control (Fig. 1C), but this difference was not significant (p = 0.068). In addition, LPA significantly increased the gene expression levels of IGF2R, PCNA and CDH1 when compared to control levels (Fig. 1D). In contrast, LPA did not alter the gene expression levels of SLC2A1, DNMT3A or DSC2 (Fig. 1D).
LPA significantly increased the numbers of total cells (TC) and ICM cells when compared to the corresponding numbers of the control group (Figs. 2A and 2B). LPA did not influence the trophectoderm (TE) cell number or the ICM-to-TE cell ratio (Fig. 2B). However, the EdU-positive cell number and the EdU-positive-to-TC ratio were significant higher in the LPA treatment group versus the control group (Figs. 2C and 2D).
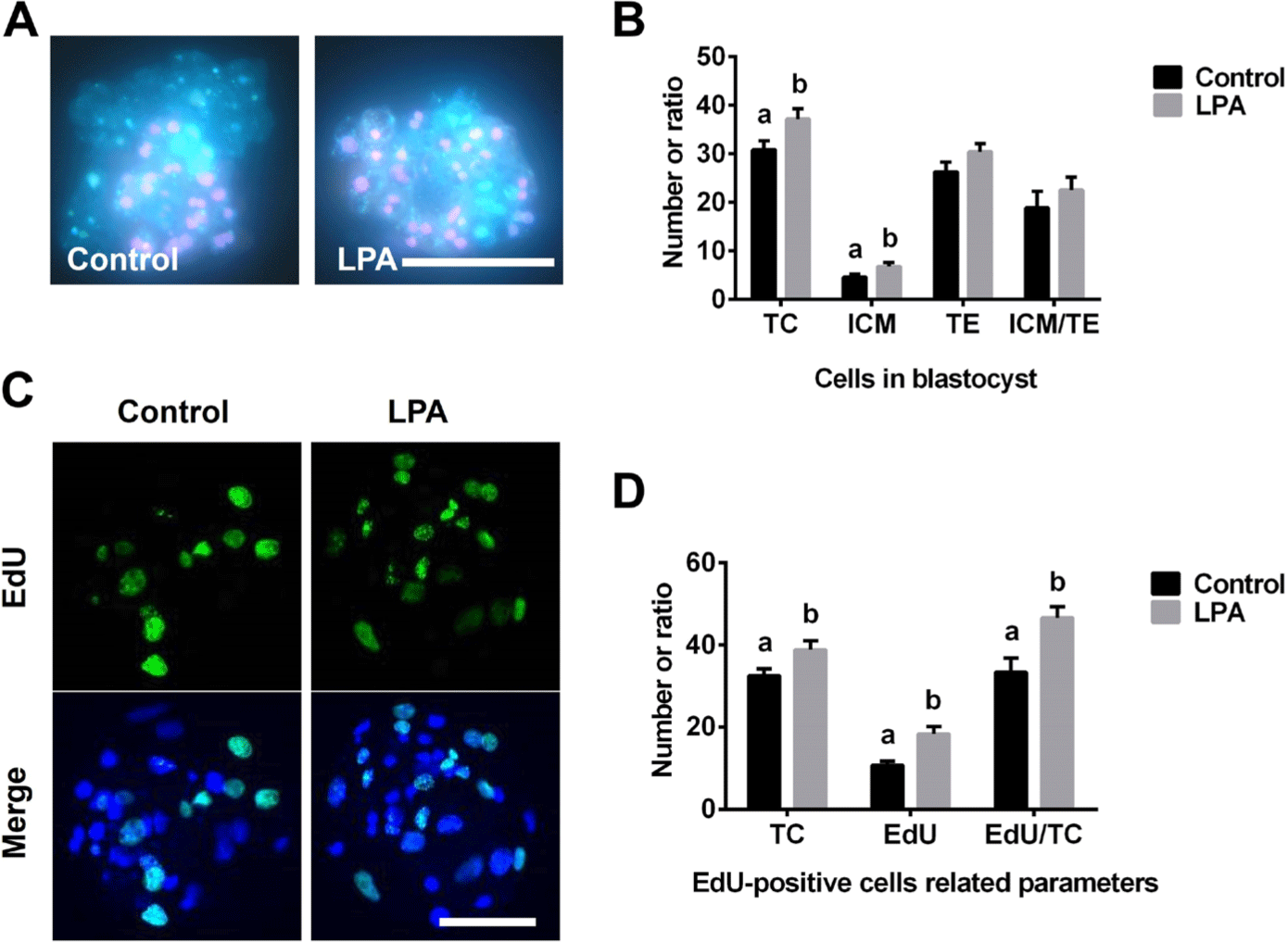
LPA significantly reduced the level of ROS generation (Fig. 3A), increased the mitochondrial membrane potential (Figs. 3B and 3C), and decreased the apoptosis rate (numbers of TUNEL-positive cells/numbers of TC; p < 0.05) compared to the corresponding values for the control group (Figs. 3D and 3E). The expression levels of the pro-apoptotic genes, BAX and CASP3, were significant lower (p < 0.05) (Fig. 3F) whereas the expression level of the anti-apoptotic gene, BCL2L1, was upregulated (p < 0.05) (Fig. 3F) in the LPA treatment group compared to the control group.
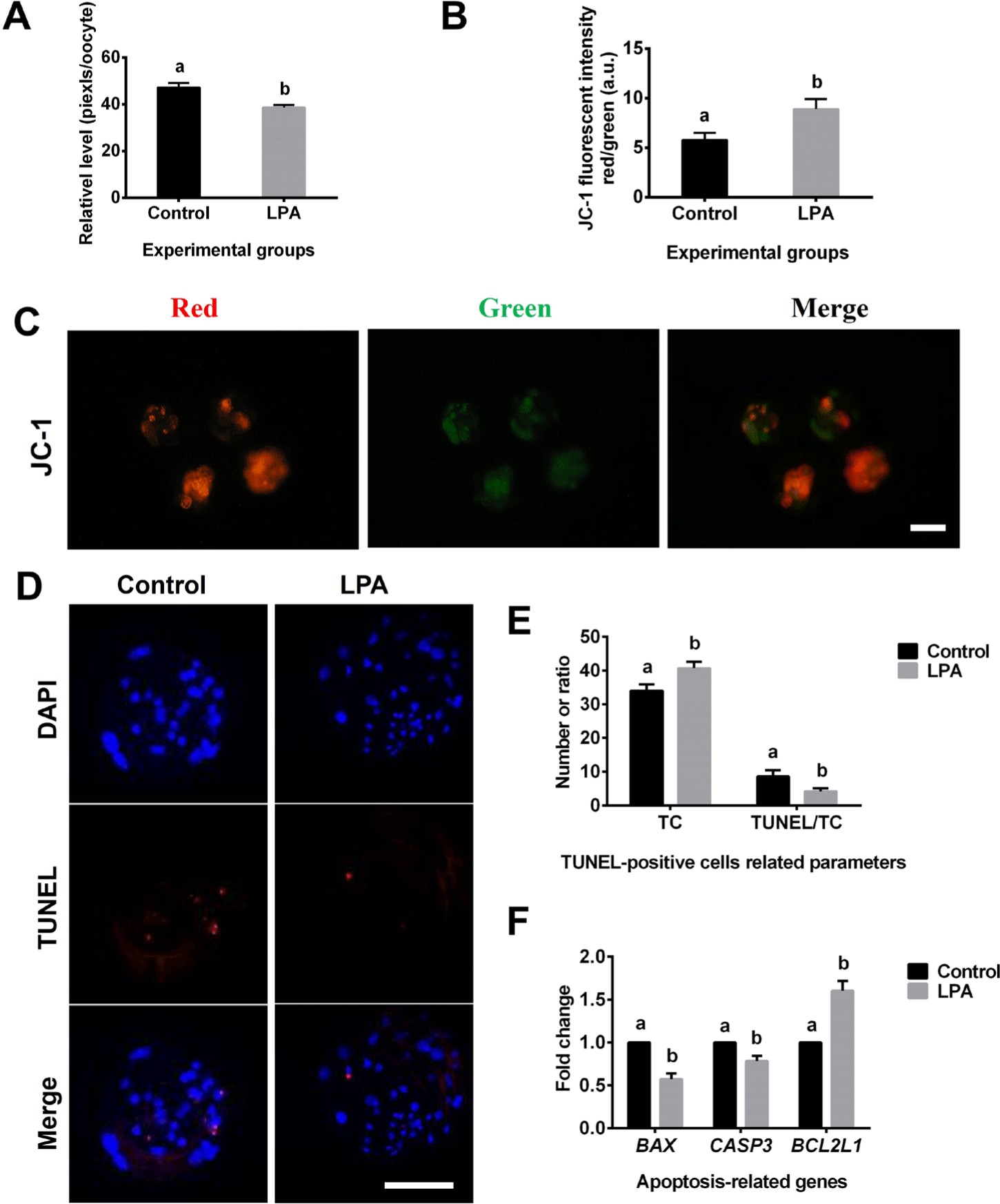
Fluorescence staining showed that the Oct4 expression level was significantly higher in the LPA treatment group than in the control group (Figs. 4A and 4B). In addition, LPA significantly increased the gene expression level of Oct4 when compared to the control (Fig. 4C). However, LPA did not influence the gene expression levels of Sox2 and Nanog in porcine SCNT-derived blastocysts (Fig. 4C).
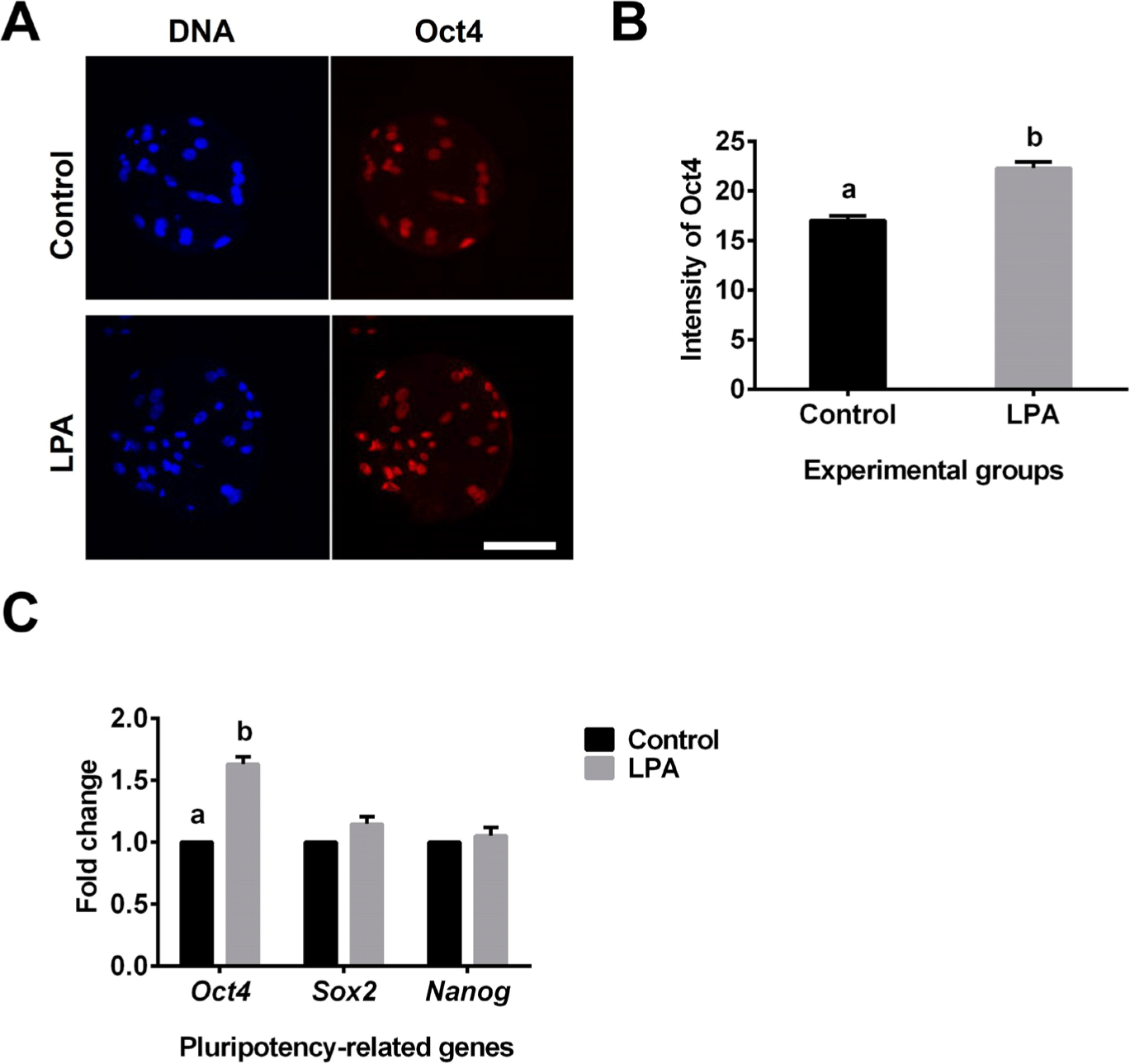
DISCUSSION
Previous studies showed that LPA could improve porcine PA- and IVF-derived embryos [23,24,31]. Our present results newly demonstrate that LPA supplementation of a chemically defined PVA-based medium (PZM-4) could improve the development and quality of porcine SCNT-derived embryos.
The number of cells in the blastocyst is an important parameter for embryo quality, as blastocysts with numerous cells possess greater cell proliferation and better implantation potential after embryo transfer [32]. The ability of LPA to increase cell proliferation was previously reported in a porcine preadipocyte cell line [19], and the addition of LPA to a BSA-based undefined medium was shown to increase the cell numbers in porcine PA and/or IVF blastocysts [24,31]. In the present study, we found that LPA increased the total, ICM and EdU-positive cell numbers in porcine SCNT-derived blastocysts. Thus, we speculate that the ability of LPA to positively influence porcine cloned embryo development and quality might at least partly reflect its ability to improve the cell proliferation potential.
The timing of cleavage and blastocyst formation can be as indicator of developmental potential and quality of embryos [33,34]. A previous study showed that early-cleaved embryos have greater potential to develop to blastocysts, compared to late-cleaved embryos [35]. In addition, early-formed blastocysts (formed on day 6) were found to be of generally higher quality than late-formed blastocysts (day 7 or 8) in pig [32]. LPA has been shown to facilitate the compaction of embryos in mice [36] and accelerate blastocoel formation in pig [23]. Here, we found that the proportion of early-cleaved embryos (at day 2 of culture) and early-formed blastocysts (at day 6) were significantly higher in LPA treatment group than the control group, indicating that LPA accelerated cleavage and blastocyst formation in porcine SCNT embryos.
It is well known that the utilized in vitro culture conditions can dramatically alter the gene expression patterns of embryos [32]. In the current study, we evaluated several embryonic development-related genes (IGF2R, SLC2A1, DNMT3A, PCNA, CDH1 and DSC2) after LPA treatment. We observed that LPA did not significantly influence the gene expression levels of SLC2A1, DNMT3A and DSC2, indicating that these genes may not participate in the activities of LPA on porcine embryos. However, the expression levels of IGF2R, PCNA and CDH1 genes in porcine SCNT embryos were significantly changed after LPA treatment. Among them, IGF2R (insulin-like growth factor 2) has been shown to play very important roles in fetal growth and placental function in mammals [37]. CDH1 (cadherin 1), which is a classical member of the cadherin superfamily, is responsible for intercellular connectivity [38]. Our observation that LPA significantly elevated the expression levels of IGF2R and CDH1 is consistent with previous reports that LPA significantly increased the gene expression levels of IGF2R in bovine embryos [25, 29] and that of CDH1 in porcine embryos [23]. Proliferating cell nuclear antigen (PCNA) is involved in the DNA replication and repair machinery that can be used to evaluate embryonic development [39]. Here, we found that LPA significantly increased the gene expression of PCNA, which would logically contribute to the ability of LPA supplementation to improve the quality of porcine cloned embryos.
Apoptosis occurs during the preimplantation embryo development of in vitro- and in vivo-produced embryos and may contribute to embryonic loss [40]. ROS and mitochondrial membrane potential can play essential roles in apoptosis. ROS can directly induce oxidative injury or trigger a mitochondrial permeability transition in cells [41]. Here, we found that the ROS level and mitochondrial membrane potential were significantly lower and higher, respectively, in the LPA treatment group compared to the control group. This suggests that LPA could effectively reduce oxidative stress and maintain the mitochondrial membrane potential in porcine SCNT-derived embryos. Furthermore, and consistent with previous reports that LPA can reduce apoptosis in the embryos of many species [15,23–26], we found that LPA significantly reduced the rate of apoptosis in porcine SCNT-derived blastocysts compared to the control. Similarly, previous reports found that the apoptosis index was decreased in LPA-treated porcine PA- and IVF-derived embryos [23,24]. In terms of apoptosis-related gene expression, LPA supplementation during in vitro culture was previously reported to significantly increased the gene expression of BCL2L1 and/or reduce those of BAX and CASP3 in bovine IVF embryos [29] or porcine PA [23] and IVF [24] embryos. In the present study, LPA supplementation was found to significantly increase the gene expression of BCL2L1 gene and reduce those of BAX and CASP3 in porcine SCNT-derived embryos. These data suggest that LPA blocks early apoptosis during porcine SCNT embryo development by modulating the expression of BCL2 family genes.
The blastocyst includes TE and ICM cells. TE cells will form extraembryonic tissues, whereas ICM cells give rise to the fetus. In the ICM cells of the blastocyst, the transcription factor Oct4 collaborates with Sox2 and Nanog form a circuit that maintains pluripotency [42–44]. These factors are usually involved in transcription regulation during early preimplantation embryo development and cell differentiation [45]. Thus, the expression levels of these factors can often be used as indicators of embryo quality and development. In the pig, endogenous Oct4 expression is maintained in all nuclei of the blastocyst, Nanog is not detected in any nucleus of the blastocyst [46], and Sox2 is specifically expressed in ICM cells of porcine blastocysts [43]. Our immunostaining results confirmed that Oct4 is expressed the TE and ICM cells of porcine blastocysts. Notably, high-level expression of Oct4 in porcine cloned embryos was previously suggested to be important for improving embryo quality and cloning efficiency [46]. In the present study, LPA significantly increased the expression level of Oct4 compared to that seen in the control group. Thus, the ability of LPA to improve the quality of porcine cloned embryos might be partially due to its upregulation of Oct4.
In conclusion, our results collectively suggest that LPA can improve the development of porcine SCNT-derived embryos in terms of reducing apoptosis and enhancing blastocyst formation, cell proliferation and pluripotency.